Abstract
Purpose
Micropollutants in the aquatic environment pose threats to both ecosystems and human health. Traditional wastewater treatment plants (WWTP) reduce some micropollutants, especially those who adhere to sludge or suspended matter. The hydrophilic micropollutants, on the other side, which may be non-biodegradable and resistant to UV-treatment etc. are typically transported untreated into the water recipients. This paper contains a literature study on the state of the art of advanced wastewater treatment technologies for reducing micropollutants such as pharmaceutical degradation products, personal care products, surfactants and industrial chemicals including heavy metals.
Design/methodology/approach
This literature study is completed using the most extensive and expansive literature database in the World to date, Google Scholar (GS). Published papers in recognized scientific journals are sought out in GS, and for relevance for this literature study, papers published here from 2016 and onwards (the last 5 years) have been chosen to eliminate irrelevant studies.
Findings
The result of the study is that there are many promising technologies on the market or emerging; however, no one solution treats every micropollutant equally well. Since advanced technologies often require expensive investments for municipalities and companies, it is important to identify which micropollutants pose the highest risk towards human health and the environment, because choosing systems to eliminate them all is not economically wise, and even choosing a system combining the existing technologies can be more expensive than states, municipalities and private companies are capable of investing in.
Research limitations/implications
The research is limited to published papers on GS, which may omit certain papers published in closed databases not sharing their work on GS.
Practical implications
The practical implications are that practitioners cannot find go-to solutions based on the conclusions of the research and thus need to use the results to investigate their own needs further in order to make the wisest decision accordingly. However, the paper outlines the state of the art in advanced wastewater treatment and explains the benefits and downsides of the technologies mentioned; however, more research in the field is required before practitioners may find a proper solution to their specific issues.
Social implications
The social implications are that the consequences of introducing a removal of micropollutants from the water environment can ultimately effect the citizens/consumers/end-users through added costs to the tariffs or taxes on advanced wastewater treatment, added costs on everyday goods, wares and products and added costs on services that uses goods, wares and products that ultimately produces micropollutants affecting the water environment.
Originality/value
This paper presents a much needed state of the art regarding the current advanced technologies to mitigate micropollutants in wastewater. The overview the paper provides supports politics on national as well as international levels, where larger unions such as the EU has stated that advanced wastewater treatment will be the next step in regulating pollutants for aquatic outlet.
Keywords
Citation
Lauesen, L.M. (2022), "Advanced wastewater treatment of micropollutants – state of the art", Technological Sustainability, Vol. 1 No. 2, pp. 101-120. https://doi.org/10.1108/TECHS-09-2021-0007
Publisher
:Emerald Publishing Limited
Copyright © 2021, Emerald Publishing Limited
Introduction
Focus on water pollution from sewer overflow, agricultural drainage as well as from treated municipal wastewater dominates the pollution discourse in aquatic environments (Souza and Féris, 2016). Research in micropollutants removal technologies (i.e. advanced wastewater treatment) has mainly taken place in industrial sectors such as hospital wastewater treatment facilities and has been fairly neglected as an issue in traditional municipal treatment plants because of the financial investment it requires on top of the nutrients removal technologies.
Micropollutants are of increasing public importance because of their nature and negative side effects affecting the environment, the wildlife, ecosystems and human health as well (Tang et al., 2020a, b, c; McEachran et al., 2016). Yet, the highest concentrations of micropollutants are found in the group of pharmaceutical degradation products, which for municipal wastewater treatment plant (WWTP) influents vary between >0.001 and <100 µg per liter in average Worldwide (see also Lindholm-Lehto et al., 2016).
This paper presents a literature study on advanced wastewater technologies to treat micropollutants before outlet to the aquatic environment with a special focus on pharmaceutical degradation products, personal care products, surfactants, industrial chemicals including heavy metals and the state of the art of advanced wastewater treatment technologies to remove these micropollutants.
Methodology
This literature study is completed using the most extensive and expansive literature database in the World to date, Google Scholar (GS) (Halevi et al., 2017). However, when using this omnipotent source of scientific literature search, certain precautions must be and has been made. Citation counts, patents and citations are not used as parametric source, since GS is not limited to scientific approval, and for clinical results, many non-peer-reviewed papers can be found in GS, which is not reliable as a primary source. Thus, published papers in recognized scientific journals are sought out, and for relevance for this literature study, papers published here from 2016 and onwards (the last 5 years) have been chosen to eliminate irrelevant studies.
Search words used in GS (5. Nov 2020)
#1 “Technologies to treat pharmaceutical compounds in wastewater” (24,800 results)
#2 “Pharmaceutical compounds in municipal wastewater” (24,900 results)
#3 “Pharmaceutical compounds in municipal wastewater + mass balance” (19,500 results)
#4 “Ozonation processes for micropollutant removal” (9,910 results)
#5 “Hydrogen peroxide processes for micropollutant removal” (6,290 results)
#6 “Fenton processes for micropollutant removal” (6,450 results)
#7 “Catalytic wet peroxide oxidation + micropollutants” (1,280 results)
#8 “Heterogeneous photocatalysis + micropollutants” (3,790 results)
#9 “Sonolysis and microwave technologies + micropollutants” (168 results)
#10 “Moving Bed Biofilm Reactor + micropollutants” (1,260 results)
#11 “Costs of advanced wastewater treatment for micropollutants” (15,100 results)
The selection for this paper was chosen out of headlines and abstract reading of the most relevant papers, where GS take in consideration the chosen wording line in terms of sorting mechanism as well. Most papers were thus chosen from the first 10 pages of each search word.
Furthermore, researchers from the Danish Technical University (DTU), dr. Kai Tang and professor dr. Henrik Rasmus Andersen, were so kind to provide relevant papers especially on MBBR technologies. Similarly, Water & Waste Denmark has provided research results from laboratory and literature studies made from the consulting company Veolia/Krüger and DTU.
Literature study
Municipal wastewater is typically treated with biological and in some instances chemical processes. Most municipal WWTPs have a form of active-biological treatment with oscillations between aerobic and anaerobic cycles, which remove organic compounds and nutrients such as nitrogen and phosphorous complexes (Tang et al., 2020a, b, c). In some facilities, a chemical process is added if certain outlet requirements cannot be met, or if certain difficult inorganic compounds need to be removed. Most nations have outlet requirements for COD (chemical oxygen demand), TN (total nitrogen), TP (total phosphorous) and SM (suspended matter, particles).
Since 1970s, the establishment of WWTPs has increased Worldwide, and most of the designs of them are variants of the active-biological design. However, in some cases, where industrial outlets represent a significant amount of the wastewater, advanced treatment technologies such as ozone or hydrogen peroxide treatments are applied as well. That can be the case if the wastewater consists of strongly bound organic or inorganic chemical compounds such as enzymes from detergents production facilities, or when large amounts of pharmaceutical degradation products are present in the wastewater. However, these advanced technologies are yet exemptions to be seen.
Besides regulated outlets of treated wastewater, the solids, such as the sludge, are used for many different purposes. Some is used as fertilizer on farmland if requirements are adequate, and some is used for biogas production. A part may be containing such high amounts of micropollutants that have to be processed in a controlled facility such as a biogas plant.
In some nations, treated and/or untreated wastewater is allowed for agricultural irrigation on farmlands or in forests (McEachran et al., 2016). Especially in areas of severe drought periods and water shortage, treated wastewater is a substitute for missing freshwater resources. Residues are here found in groundwater aquifers as well as surface water bodies.
Micropollutants
Micropollutants are often referred to in groups of flame inhibitors, surfactants, pharmaceuticals, personal care products, endocrine disruptors, natural and synthetic hormones, illicit drugs, gasoline additives and heavy metals. They are all known to cause negative environmental effects, such as short-term and long-term toxicity and antibiotic resistance of microorganisms (Garcia et al., 2007; Thuy and Nguyen, 2013; cited from Souza and Féris, 2016, p. 871). These micropollutants have been found in both aqueous and suspended solids as well as in mixed liquor, secondary, and final effluents, and in waste and return sludge fractions (Brown and Wong, 2018, p. 774).
Many micropollutants have a bioaccumulating effects – i.e. they disperse through the food chain. Some are polar, which means their bioaccumulating effect stays in the aquatic environment. When found in receiving water bodies, some micropollutants adhere to sediments, others are more water dissolvable and end up as uptake in biota (smaller living animals), and only part of them are degradable according to biological or photo degradation (sun light). The effects of micropollutants on biota have been shown similar to human medical treatment effects, for instance; anti-depressants have side effects such as inhibiting estrogen effects, impair animal physiology, vital organs and reproduction, mutations and antibiotic-resistant bacteria. Their genotoxicity on small living biota is still in its early stage of research, and data about the environmental effects of pharmaceuticals and other micropollutants are thus still limited (Lindholm-Lehto et al., 2016, p. 7986). Similarly, some anti-inflammatory drugs such as diclofenac have shown to be lethal to zebra fish embryos and their teratogenicity (neural defects) is well described in the literature (Capodaglio et al., 2018).
Biological treatments are often not able to completely remove for instance antibiotics and phenolic endocrine disrupting compounds in municipal WWTPs (Spataro et al., 2019). This is also seen when treated municipal wastewater is used for farmland or forestry irrigation, where soil and forest systems filter certain nutrients from wastewater to acceptable criteria levels before reaching surface water sources. Here residues from pharmaceuticals and personal care products can be re-detected in surface waters after irrigation, especially micropollutants from antibiotics, antimicrobials, prescription and non-prescription drugs (McEachran et al., 2016, p. 901).
Furthermore, when wastewater is used for crop irrigation, the transfer of pharmaceuticals and personal care products have been found in studies directly on the crops afterward (e.g. Riemenschneider et al., 2016), some with no risk and others with high risks to the environment. Similarly, pharmaceuticals and their residues have been detected in surface waters where concentrations in the influents and effluents from WWTPs can range from hundreds of nanogram per liter to microgram per liter and vary with the season – higher concentrations in the winter season, because of the less degradation with less UV radiation in the winter as well as lower temperature compared to the summer season (see Lindholm-Lehti et al., 2016, p. 7985).
Many pharmaceuticals and their degradation products have little metabolism throughout a standard treatment process in municipal WWTPs. Some (such as broad-spectrum antibiotics as macrolides (Lin et al., 2018)) adhere to suspended solids and sludge, while others are found stable in mixed liquor depending on the chemical's specific hydrophilicity (Brown and Wong, 2018). No further effect is seen through the UV exposure treatment compared to the other processes in the WWTP.
Some pharmaceuticals, however, can be reduced through conventional and UV-treatment in the WWTP (Lin et al., 2018; Ashfaq et al., 2017; Thiebault et al., 2017). Yet, some pharmaceuticals such as for instance betablockers, antibiotics – especially the macrolides (broad-spectrum antibiotics) and NSAIDs are concentrated throughout the WWTP treatment (Březinova et al., 2018). Some antibiotics can adhere to sludge (e.g. fluoroquinolone, another broad-spectrum antibiotic) and be seen removed up to 99% in traditional WWTPs (see Guo et al., 2017), whereas opioid analgesics (morphine etc.) and their metabolites are common constituents of municipal wastewater as well as the receiving river water (Krizman-Matasic et al., 2018), which indicates that removal in WWTP is limited.
Castiglioni et al. (2018) studied more than 80 micropollutants up- and downstream 3 WWTPs with outlets to the River Lambro Basin in Milan, Italy. 37 measured substances were pharmaceuticals, and the rest consisted of illegal drugs (28), personal care products (PCPs) (4), disinfectants (DIS) (2), perfluorinated compounds (PERF) (2), alkyl- and bisphenols (Alk-BPA) (4) and anthropogenic markers (AM) (5) grouped as shown in Figure 1. The data shown below have been re-calculated by the author of this paper into the reduction through the 3 WWTPs mentioned in Castiglioni et al.'s research and depicted graphically by the author of this paper to suit this paper's purpose. The data behind Figure 1 can be seen in Castiglioni et al. (2018, p. 292, Table 2).
Castiglioni et al.'s (2018) results indicate strongly that many micropollutants are reduced in the WWTPs processes (which included UV disinfection), however PDPs such as anticancer, CNS (central nervous system), and opioid drugs as well as perfluorinated compounds (PERF) are up-concentrated in the outlets of the 3 WWTPs.
The Danish wastewater treating company BIOFOS investigated pharmaceutical micropollutants in their 7 WWTPs (without UV disinfection) in 2015 (see Figure 2).
Their results showed that some pharmaceutical micropollutants were reduced throughout the WWTP processes while others, especially anticancer, opioids and CNS drugs often were not reduced (Kayerød and Hansen, 2019).
Micropollutants in drinking water resources
Pesticides, herbicides and fungicides are more often detected in freshwater wells as a first source of find, because waterworks worldwide are regulated towards many chemical residues limitations, which it must live up to before freshwater can be distributed to consumers. One of the recent issues in the EU regarding freshwater quality is derivatives or residues of pesticides, herbicides and fungicides used many years ago in agricultural farming and management (Lauesen and Bjerre, 2020).
Another source of find of pesticides, herbicides and fungicides have been studied in the US in areas of water shortage from drought, where some states reuse stormwater and eutrophic water and advanced treated wastewater as alternative sources for freshwater generation out of treated secondary water sources (Glover et al., 2019). One highly toxic micropollutant is called NMOR, which is a byproduct which is formed by a reaction of a secondary amine (morpholine, monochloramine and dichloramine) found in household products such as laundry detergents, dishwashing soap, dyes, but also in the production and use of pharmaceuticals optical brighteners, waxes/resins for food paper coatings, polishes, rubber-processing chemicals, corrosion-inhibitors for boiling waters, in the production of ionic liquids and many others.
Animals exposed to NMOR have shown damages and tumors in their livers and kidneys, which is why recommendations from Germany and the US both for NDMA as well as NMOR is to regulate water reuse with 10 µg/L, whereas Australia has set a limitation on 1 µg/L for water reuse guidelines. NMOR is hardly biodegraded and thus not reduced in traditional WWTPs, and only advanced technologies such as reverse osmosis (RO) combined with UV-treatment, chlorination, ozonation, granulated active carbon adsorption (GAC) and hydrogen peroxide (H2O2) has been effective in reducing it (Glover et al., 2019, p. 307). See under “Advanced technologies for removal of micropollutants”.
NDMA is another toxic byproduct of the fungicide based on N,N-dimethylsulfamid (DMS), which has shown carcinogenic side effects much worse than the original product DMS. Schmidt and Brauch (2008) found that DMS is not reduced in aerobe filters, and that ferric chloride and poly-aluminum chloride do not significantly reduce DMS, however GAC has a significant effect, but because of rapid adsorption, the GAC filters are fed up in short time, which makes this technology extremely expensive, because filter material must be changed after a few months in operation.
In Hvidovre municipality in Denmark, the costs of using GAC to remove DMS have added 5–6 DKK (approx. 0.6–0.7 GBP) per m3 treated freshwater (Krüger, 2019). Other tests in Denmark have showed that nano-filtration in membranes reduced DMS 75–80%; however, with this technology, a large amount of the freshwater must be directed to the sewer systems, which indicates a severe water spill. Schmidt and Brauch (2008), Kaarsholm (2019) and Hofman-Caris et al. (2015) have further evaluated the following chemical reduction technologies for DMS (Krüger, 2019):
Chloridedioxide (ClO2): No significant reduction.
Hydrogen peroxide (H2O2): No significant reduction.
Caliumpermangane (KmnO4): No significant reduction.
UV: No significant reduction.
Hypochloride acid (HOCl): DMS is reduced completely to chloride. Byproducts are not identified. If ammonium is present in the water, NDMA is produced and carciogenic trihalomethanes are produced.
Ozone: DMS is reduced completely, but NDMA is produced with bromide in the water.
UV/H2O2/GAC: DMS is 100% reduced, and NDMA is reduced >95%
Advanced technologies for removal of micropollutants
Although traditional WWTPs reduce some micropollutants from effluents, the substances removed are rarely mineralized nor digested into non-toxic compounds ultimately (Capodaglio et al., 2018; Klavarioti et al., 2009). As we have seen earlier, they are typically bound to sludge or suspended solids, which in the traditional WWTP are dealt with either by biogas production or distributed on farmlands. In the latter case, the micropollutants and their metabolites or byproducts may be further released to either crops or groundwater aquifers eventually. Furthermore, it is often unknown to what degree metabolites or byproducts are even more harmful to the environment and humans and animals in the food chain than their original chemical formula. Thus, studies of advanced technologies have been made to not only remove micropollutants from the water phase, but also to eliminate (i.e. break down) substances into less harmful metabolites.
Operating advanced technologies
Advanced wastewater treatment technologies on the market are still relatively few and new, which means that although some plants have shown good results, the field of micropollutants removal and studies specifically on these compounds is limited, and many researchers point to the fact that even operating advanced technologies are still not fully explored.
There are various new technologies on the market, of which the majority is tested in laboratories and fewer in situ in pilot projects, of which some have become permanent installations in WWTPs. The technologies can be divided into six overall process categories: ozone-; peroxide-; Fenton-; photo-; electro; and membrane processes (see Figure 3).
Ozone/hydrogen peroxide reactors
Ozone (O3) is a very effective oxidative abatement for certain classes of xenobiotic micropollutants. Its willingness to react with other chemicals in releasing the third oxygen atom has shown good results even with hardly reacting or degrading molecules (for instance enzymes) and strong chemical bonds. However, one main challenge with ozonation is the limitation of O3, because this molecule prefers to become O2, which is a stronger chemical binding than O3, and thus ozone reactors work through enforcement of natural O2 to become O3, after which the O3 immediately must come in contact with other reactants, or else, it will form O2 again. Thus, ozone reactors consume high amounts of energy for its processes, which implies a high amount of CO2 emission as well, which is one of its main limitations.
Despite the effectiveness of ozonation, some micropollutants lack electron-rich functional groups, which means they cannot be oxidized efficiently with ozone alone and thus need a further production of hydroxyl radicals (OH) to initiate reactivity (for instance through combination of O3/UV or O3/H2O2 reactors as well as with activated carbon filters). O3 is typically first consumed by organic matter or compounds in wastewater, so for micropollutants to be targeted, a combination of advanced reactors is often needed (Vatankhah et al., 2019; Krüger, 2019).
In a pilot scale study, Vatankhah et al. (2019) studied 13 selected micropollutants in wastewater after it had been treated in traditional WWTPs and processed through ozonation reactors and granular activated carbon (GAC) filters in different combinations. They studied the effect on adding ozonation only; adding GAC only; and adding a combination of ozonation and GAC after traditionally WWTP treatment, respectively (see Figure 4).
Ozonation alone (after traditional WWTP treatment) showed a significant reduction of micropollutants such as antibiotics (Sulfamethoxazole and Trimethoprim), anti-inflammatory (Naproxen) and lipid regulator (Gemfibrozil), however more varied results regarding CNS drugs (Carbamazepine, Fluoxetine, Primidone and Meprobamate), disinfectants (DIS = Triclosan and NMOR = by product), and less reduction in pesticides (DEET), sweetener (Sucralose) and low reduction in reducing agents (TCEP).
Carbon filter alone (after traditional WWTP treatment) did not have similar reduction possibilities; however, the combination of ozonation and carbon filtration (after traditional WWTP treatment) showed a higher reduction than ozonation alone for the contrast agent, pesticide, sweetener and reducing agent (Vatankhah et al., 2019).
In Sweden, Antoniou et al. (2013, p. 42) studied 42 pharmaceuticals treated with ozonation alone after traditional WWTP processes in six different places. They found with varying ozone doses to reach 90% reduction of all substances. Furthermore, they found that 15 products were easily degradable; 19 were moderately degradable, and 8 were recalcitrant towards ozonation.
Finally, no particularly groups of medicine stood out in their study: antidiabetics, lipid regulators, cardiovascular, antibiotic, CNS drugs, antihistamine, opioids, anti-inflammatories and gastrointestinals were found easily degradable, moderately degradable and recalcitrant towards ozonation. Anticancer, however, was recalcitrant towards ozonation (Antoniou et al., 2013, p. 47, see table 2).
Fenton processes
Fenton processes are also emerging technologies, which combined with other advanced technologies can remove certain micropollutants. Fenton processes use hydrogen peroxide as oxidant and ferrous ions as catalysts and have been used to treat micropollutants such as pharmaceuticals, miscellaneous industrial chemicals, personal care products, endocrine disrupting compounds and fermented water combined with different other advanced treatment technologies such as UV, ultrasonic, electric or microwave technologies and various biological treatment processes (Huang et al., 2017). The combination of Fenton processes and biological treatment has been used to reduce biodegradable compounds as well as non-biodegradable micropollutants.
For instance, the group called endocrine disrupting compounds (pesticides, herbicides, fungicides, industrial compounds such as bisphenols, phthalates, PCBs, benzene esters, dyes etc.), are often resistant to biological treatment, however can be degraded to a high degree by Fenton processes and biological treatment in combination (see Oller et al., 2007; 85% reduction of pesticides and 92% reduction of dissolved organic carbon) (Huang et al., 2017, p. 1605).
Fenton processes have shown high efficiency in studies since the beginning of the Millennium (see Huang et al., 2017) however mainly in highly concentrated industrial WWTPs and not particularly in municipal WWTPs, where the micropollutants are less concentrated. Fenton processes are relatively expensive to install because of their high energy consumption for catalyzation etc. however, whenever used, these technologies combined with bioreactors can also substitute traditional processes such as nitrification/denitrification tanks, which fill up most of traditional municipal WWTPs (see Nousheen et al., 2014; Fernandes et al., 2014).
Catalytic wet peroxide oxidation
A newer tested technology since the late 1990s is called catalytic wet peroxide oxidation, which utilizes two components in reducing micropollutants not reactive to traditional WWTP processes: a catalyst (typically iron-based), an oxidizer (H2O2 – peroxide) and the original water phase in which pollutants exist. It is considered a low cost technology, because it does not require lamps (like UV), does not create or significantly reduces sludge generation compared with Fenton processes), and the process can be achieved in normal atmospheric temperature and pressure (Márquez et al., 2018).
A Spanish project, REMTAVARES (Gomes et al., 2016; Ribeiro et al., 2016), has in laboratory-scale tested catalytic wet peroxide oxidation on the antibiotic sulfamethoxazole, which is a xenobiotic compound that does not react with traditional WWTP processes but requires additional oxidation. The test was made on urban wastewater as well as hospital wastewater with a very complex mixture of both natural as well as other xenobiotic compounds. The results indicated that an absorbance of the tested sulfamethoxazole was detected of up to 74.9% in the hospital wastewater under normal atmospheric pressure and in room temperature. Furthermore, the results showed indication of an even more efficient procedure under higher temperature, which was not tested in the project, however (Gomes et al., 2016, p. 2).
A Finnish literature study on catalytic wet peroxide oxidation processes in real wastewater published in 15 publications out of more than 250 articles on the technology (mainly laboratory scale tests like the above mentioned Spanish study), however, concluded that in real wastewater, catalysts can be leaching or be deactivated due to mechanical and thermal degradation, poisoning, fouling, etc.; that continuous fixed bed reactors were not extensively studied for treatment of real wastewaters; the following toxicity of wastewaters treated by CWPO is not studied thoroughly; but that CWPO can be regarded as economically viable for wastewater treatment, especially when conducted at ambient temperature and natural pH of wastewater (p. 673).
UV irradiation
Ultraviolet irradiation is one of more known photolytic instruments that efficiently kills bacteria and organic compounds. It is often used in both waterworks for drinking water production as well as in WWTPs. UV in combination with other advanced technologies has many uses especially in the reduction of micropollutants that are recalcitrant towards normal urban WWTP processes. It is used as a tertiary treatment after traditional biological and chemical wastewater treatment in many WWTPs around the world as a first step towards advanced wastewater treatment.
Heterogenous photocatalysis
Ozonation assisted with photocatalysis from UV light or sunlight is often referred to as heterogenous photocatalysis when it is assisted by a photocatalyst as well – typically titania (Herrmann, 1999). It is heterogeneous because there are two different effects working together: light and a photon efficient absorbant metal as co-reactor.
Herrmann refers to a long list of micropollutants (e.g. Blake, 1997) that in the literature has been shown to be capable of removal through photocatalysis alone (without ozonation), which includes alkanes, aliphatic alcohols, alkenes, aromatics, phenolic compounds, amides, acids, surfactants, herbicides, pesticides and dyes (see Herrmann, 1999, p. 121). Assisted with ozonation, a large range of inorganic micropollutants can be removed as well, such as heavy metals, sodium, potassium and calcium.
Silva et al. (2019) found that UV light-assisted photolytic (light alone, e.g. UV-A/UV-C and) ozonation processes and photocatalytic (light and a photon efficient absorbant metal reactor, UV-A/TiO2 [titanium-dioxide] and) ozonation following traditional wastewater treatment created up to 54% and between 105 and 127% higher degradation of the recalcitrant and ozone-resistant pesticide acetamiprid than ozonation alone, respectively.
Electrochemical oxidation
Instead of photolysis, electric creation (anode-cathode process) of OH-radicals is an alternative way to obtain similar effects to attract micropollutants that do not adhere to or are recalcitrant to traditional WWTP processes. Electrochemical Fenton process is one methodology, and it uses electrons as reagent and creates hydrogen peroxide (H2O2) in situ, and if iron is presented in the wastewater, this is a strong co-catalyst as well. The process can reach high degrees of mineralization and degrading a wide array of contaminants, however mainly organic particles, for instance pesticides and landfill leachates (Mousset et al., 2016). However, the process requires energy for aeration and oftentimes external supply of oxygen (O2), which is not the cheapest solution.
Sonolysis and microwave technologies
As known from household appliances, microwave offers special features such as fast, uniform, selective and controlled heating that result in a rapid reaction rate for chemical reactions, when combined with other oxidation processes (Verma and Samanta, 2018). Sonolysis, on the other hand, involves ultrasonic waves that break chemical bonds or form radicals from existing compounds in their proximity by using ultrasound. In other words, sonolysis is an effective oxidator.
For instance, Fraiese et al. (2019) showed that sonocatalysis (combined with TiO2 (titanium dioxide) was even more effective on certain recalcitrant micropollutants such as the pharmaceuticals of sulfamethoxazole, diclofenac and carbamazepine than were ozonation and photocatalysis, respectively. However, the sonocatalysis results showed no significant variation occurred as dissolved organic carbon contents, which indicates an inefficient mineralization and presumably a formation of other products from the degradation of other micropollutants.
Another study carried out by Choi et al. (2019) (p. 194) showed that the potential in using fluidic sonolysis with a superhydrophobic Si (silicon) nanowire structured channel, providing an air-water interfacial cavitation for efficient generation of OH radicals without any additional energy inputs or additives removes various organic pollutants including 4-chlorophenol, bisphenol A, carbamazepine and propranolol completely within 12 min, as opposed to hours needed by other advanced technologies.
Risks identified in advanced oxidation processes
The above technologies have been studied in the last two decades, which means that the risks following some of the processes have also been identified to a certain degree. These risks concern mainly the topic of degradation products created from the oxidation processes (i.e. ozonation, peroxide, UV-treatment and the combination hereof), and sometimes even more toxic compounds are created. The issue at stake is that oftentimes, the researchers do not know the xenobiotic toxicity of the degradation products yet, because they are so new, that they have not been studied in the environment yet.
For instance, Wang et al. (2018) studied the toxicity of UV-based advanced wastewater treatment alone and in combination with peroxide (H2O2). They found that most pollutants treated with UV light or UV and H2O2 create byproducts, of which 81% are toxic and 50% more toxic than the parent pollutants; others were sensory-unpleasant (unwanted color, taste and odor properties) or assimilable (look like carbon and proliferate microorganisms and disturb the bio-balance in the wastewater), however, all of them pose a risk to human health and/or an ecological risk. Furthermore, they found that toxic byproducts may be generated from hydroxylation, dealkylation, decarboxylation and deamination; that “the assimilabilities of treated pollutants were generally >5 times (64% probability) and 1–5 times (85% probability) that of the parent pollutants for organic micropollutants and DOM [dissolved organic matter], respectively”; and that ozonation and chlorination of organic pollutants and the subsequent metabolism of microorganism may result in sensory-unpleasant byproducts (Wang et al., 2018, p. 122).
Similarly, Herrmann (1999) mentions that UV-A/TiO2-ozonation processes may be very effective in reducing organic and inorganic micropollutants, however the “efficiency of photocatalysis with halogenated compounds is sometimes very toxic for bacteria in biological water treatment” (p. 128).
Emerging advanced technologies
Photocatalytic membrane reactors
As shown in the above literature study, anticancer drugs and their metabolites do not react to traditional biological or chemical treatment in WWTPs and neither to UV-disinfection. Photocatalytic membrane reactor (PMR) is an emerging technology that has been tested in Spain on 30 cytostatic compounds, which are drugs designed for cancer treatment and are supposed to be persistent to all other reactions than to the specific therapeutic purpose in a patient's body (Janssens et al., 2017). Furthermore, these drugs showed to be mutagenic, cytotoxic (towards cells), genotoxic (towards genes), cyanogenic (towards microorganisms and fungi), teratogenic (towards embryo or fetus) and fetotoxic (towards embryo or fetus) and non-biodegradable.
The PMR has a high energy consumption to generate the photocatalytic process, the oxidation process and the filtration process in the membrane. However, although not so high as alternative separation processes such as UV light, ozone or hydrogen peroxide and combinations of these. The results show that there is up to 100% removal of the cytostatic compounds in the PMR reactor. Drawbacks to the PMR technology at its present stage of development is that although the cytostatic compounds are reduced, they are in fact more likely split into metabolic compounds, whose effects are still unknown in the environment (Janssen et al., 2017, p. 30). The PMR technology has been estimated to cost 20 times less than for instance ozone/UV-treatment reactors under similar operating and maintenance costs (Plakas et al., 2016), but up to 10 times as expensive than for instance powdered activated carbon adsorption coupled as Ultra Filtration membranes (Janssen et al., 2017, p. 34).
Microalgae mediated bioremediation
Microalgae mediated bioremediation includes bioadsorption, bioaccumulation and intracellular and extracellular biodegradation, and it has been well reported on laboratory scale. Microalgae have a potential not only to adhere to some pharmaceutical substances, but also to biodegradate organic compounds and yet in some settings split pharmaceuticals into metabolites due to their electric reactive abilities. However, although microalgae can reduce organic material close to 100%, it is less effective when it comes to inorganic compounds in pharmaceuticals. However, conglomerates of different algae or combinations of algae and bacteria have shown emerging potentials in reducing pharmaceuticals in WWTPs (Xiong et al., 2018). Xiong et al. (2018) have reported the newest trends in microalgae mediated bioremediation of pharmaceuticals, which is depicted in Figure 5. Mixotrophic microalgae can adapt to and grow and thrive in extreme environments such as wastewater, and these algae can shift metabolism depending on carbon and nutrition resources available.
Figure 5 is depicted with a similar x-axis as Figure 1, 2 and 4 for comparison although with two added substances: steroids and contrast agents. Figure 5 shows the reduction of pharmaceuticals in mixotrophic microalgae treatment, and the empty categories (anticancer, gastrointestinal, cocaine and metabolites, amphetamines and ketamine, cannabinoids, PCPs, PERF, Alk-BPA) are not present in the literature study of Xiong et al. However, comparing Figure 5 with Figure 1, 2 and 4 shows that mixotrophic algae reduce many of the pharmaceuticals as did the traditional WWTPs.
Some interesting differences are seen with mixotrophic microalgae, though. Xiong et al.'s literature study of the mixotrophic microalgae did not report on anticancer drugs, and in the study by Castiglioni et al. anticancer drugs were passing through WWTPs in Italy (0% reduction). CNS (central nervous system) as well as opioid drugs, however, were found in Xiong et al.'s study to have been reduced by 94% and 54–95% by mixotrophic microalgae, but in Castiglioni et al.'s study, both pharmaceuticals passed through the WWTPs. In this respect, mixotrophic microalgae is an interesting, advanced treatment option for pharmaceutical substances, although studies are still in their early phases.
Moving bed biofilm reactor and ozonation
Micropollutants, especially pharmaceuticals from cancer treatment, anti-inflammatories and drugs used in pathology medicine and for surgery, have proved among the hardest to reduce in conventional WWTPs. Most pharmaceuticals, however, are not found directly in wastewater from hospitals, but primarily from households, because more and more are treated on outpatient basis (Naturstyrelsen, 2015).
In Denmark, the pilot project MERMISS took place from 2015–2017 on Aarhus Universitetshospital (AUH Kommunehospitalet), Skejby Hospital and on WWTPS in Herning, Egå and Viby, Aarhus, respectively. 6 pilot scale Moving Bed Biofilm Reactors (MBBR) were tested here for the reduction of 22 different pharmaceuticals both at the hospitals as well as at the WWTPs (EPA Denmark, 2018).
The MBBR technology is based on slowly growing bacteria on a plastic carrier protected by a protein film, on which the bacteria can remain for an extended time in the wastewater without degrading as fast as in traditional active-sludge plants (EPA Denmark, 2018, p. 5). Together, the bacteria and protein film are called a “biofilm”.
The biofilm is bacteria gathered on a surface, which means the bacteria are the main ingredients in the biofilm. Polymers bind the bacteria and protect them. The advantage of biofilm is the long hydraulic retention time, which promotes the slow growing bacteria, which are better in digesting or reacting to compounds in the wastewater. The faster retention time in active-sludge processes means that the bacteria are moving around from process to process (in the sludge), and because traditional processes shift between aerobic and anaerobic (with or without oxygen) processes here, the bacteria have another characteristic and need to be adjusted to those different environments, which gives them a shorter life cycle. To react with the resilient substances in micropollutants, a long retention time and strong, but different bacteria are needed in separate reactors.
MBBR is thus designed with different kinds of bacteria in each reactor, which means that the specific bacteria environment is protected, nursed and adapted to the particular wastewater without risking being expelled or outnumbered by other bacteria in the process.
The combination of reactors in the MBBR-technology can consist of micro fungi, different bacteria and micro animals. The reactors in the MERMISS project were followed by an ozone reactor with hydrogen peroxide catalyst for optimization of the retention time and the degradation of the ozone depending on the micropollutants to be reduced (Tang, 2018). In the fine polishing were also a granular active carbon filtration unit and UV-treatment.
Many advanced wastewater treatment technologies have a high reduction rate for some medications e.g. painkillers including ibuprofen and paracetamol, which easily react with the bacteria in the protein film (the biofilm) up to 100%, however, in traditional WWTPS without advanced technologies, these substances are reduced as well to a high extend.
With the hardly degradable pharmaceuticals such as e.g. Diclofenac (painkiller), Venlafaxine (psychopharmaceutic), Azithromycin (antibiotic of the type macrolide), Metoprolol (cardiovascular drug) and anticancer drugs, the advanced technologies have superior qualities. In traditional WWTPs, Diclofenac is typically reduced around 20%, whereas in MBBR (and other advanced technologies) a reduction of up to ca. 80% has been seen. The Venlafaxine is a bit harder to reduce, and in traditional WWTPS no reduction is typically seen, whereas in MBBR (and other technologies) a reduction of up to 50% has been shown.
The highly resistant antibiotics such as Macrolides are too very hard to reduce, but while e.g. Azithromycin is reduced ca. 22% in traditional WWTPs, the MBBR technology has managed up to 44% reduction (and in specialized microalgae systems up to 89%). Metoprolol appears in the MBBR project as a negative reduction – which means it is concentrated, but with specialized microalgae, a reduction between 75 and 100% was seen. These specialized microalgae could probably be a part of the MBBR design as well. In the MERMISS project, however, the former pharmaceuticals were reduced in the following ozone- and GAC processes treating the hospital wastewater after the membrane processes.
The hardest pharmaceuticals to reduce are within the category of anticancer drugs, of which some – especially those used in chemotherapy – are molded to kill cells and are thus recalcitrant for biological degradation. Even oxidation can be tricky for reduction; however, photo-catalyzation has shown some effect (e.g. Janssens et al., 2019; Perreira, 2020; Kehlbert et al., 2020; Chen et al., 2019). With oxidation, there is a risk that the by products can be even more toxic than the original micropollutant as seen with the nitrosamines (NDMA and NMOR) earlier mentioned.
The pilot project MERMISS is continued in the current research project MEREFF, where new results are expected published during 2021. The researchers expect that MBBR will be able to reduce biological oxygen demand (BOD) with nitrification- and denitrification processes attached as a pre-polishing of chemical oxygen demand (COD) for following ozonation for extended fine-/post-polishing of pharmaceuticals on municipal WWTPs.
Membrane bio reactor and ozonation
Another Danish pilot-scale test on 42 pharmaceuticals in hospital wastewater with Membrane Bio Reactor (MBR) was carried out from 2014–2016 at Herlev Hospital (Grundfos, 2016). The MBR technology in the trial consisted of a biofilm on carriers made by 16 ceramic filter membranes (see Figure 6). The installation in Herlev Hospital consisted of two different setups (Line 1 and 2) with additionally two different ozonation dosages (Line 1: 6 mg and 15 mg O3/L respectively; Line 2: 7 mg and 24 mg O3/L, respectively), of which Line 2 with 24 mg O3/L had significantly better efficiency in reducing all pharmaceuticals to outlet requirements than the other setups:
Line 1: MBR – GAC – Ozone – UV
Line 2: MBR – Ozone – GAC – UV
The 42 pharmaceuticals grouped as previously show how all groups of pharmaceuticals have substances that are easily or relatively easily reduced with MBR, however in the same group there are substances that are even recalcitrant to biological degradation.
MBR alone (like the MBBR-technology) reduced pharmaceuticals such as ibuprofen (anti-inflammatory) and paracetamol (painkiller) 100%, while the harder degradable substances such as Diclofenac (painkiller) and Venlafaxine (psychopharmaceutic) were concentrated; Azithromycin (antibiotic of the type macrolides) were reduced ca. 18%; Metoprolol (cardiovascular) were reduced ca. 54%. Chemotherapy drugs were hardly reduced in MBR as well as in MBBR-technologies, however some chemotherapy substances that kills cells (e.g. Tamoxifen and Capezitabine) were reduced more than 80% with MBR technology alone. As with MBBR, the ozonation, GAC and UV after the MBR reduced the rest of the pharmaceutical micropollutants to the limits for outlets.
The costs of advanced wastewater technologies
The literature rarely dwells with the costs of advanced wastewater technologies but is rather interested in its effect. However, for states, regions, municipalities and private companies to take responsibility toward reducing micropollutants in the environment, costs are necessary to know for decision makers.
Below in Figure 7, the cost estimates of the above technologies are shown in increasing costs moving from the left to the right on the x-axis.
Conclusion
This literature study has showed the state of the art of advanced wastewater treatment technologies, and the result of the study is that there are many promising technologies on the market or emerging, however, no one solution treats every micropollutant equally well. Since advanced technologies often require expensive investments for municipalities and companies, it is important to identify which micropollutants pose the highest risk towards human health and the environment, because choosing systems to eliminate them all is not economically wise, and even choosing a system combining the existing technologies can be more expensive than states, municipalities and private companies are capable of investing in.
However, the EU commission has highlighted micropollutants as the next generation challenge and evidence of the toxicity of these substances are already present in natural water bodies such as lakes, streams, rivers and the sea, hence it is crucial to address and take responsibility of. Yet, no quick fix seems up the alley, and no single technology has gained worldwide acknowledgment as part of regular wastewater treatment such as in the 1980s, where the introduction of biological/chemical wastewater treatment with aerobic/anaerobic active sludge treatment soon became the best available technology worldwide.
Regarding micropollutants and the extreme variety and concentration it appears in from city to city, each municipality/region/state must act and decide according to its concise challenges and for themselves choose what is the most important substances to treat; how much can we afford to invest; and which technology is the best for this particular wastewater profile at hand locally.
Governmental actions: Policy-makers should consider assisting the municipalities/regions/states etc. in not only proposing or even setting limit values for micropollutants in outlets, which in some instances equals the detection limit, but relate the limit values to toxicity analyses and communicate clearly to municipalities/regions/states and even end-users (e.g. consumers) why this limit value is chosen, and which technologies are available to mitigate the challenge of removing the micropollutants. Furthermore, policy-makers should take in consideration communicating what costs are induced upon the operators (water utilities, -companies/municipalities etc.) and ultimately to the consumers via added taxes and tariffs for removing micropollutants and why.
Since micropollutants can be a severe environmental as well a human health issue on short as well as long scale, communication and full transparency in policy-making may facilitate a higher degree of acceptance among end-users/-payers in society that removing these man-produced and -used chemicals from the environment enhances the sustainability of both people and the planet.
Company actions: Company owners should consider substituting chemicals that produce harmful micropollutants with substances that produce non-harmful or less harmful micropollutants. In the case of this option is non-available, for instance hospitals treating patients with chemo-therapy medicine for curing or relieving cancer, companies should take part and invest in micropollutant removal from their industrial wastewater as an improved life cycle impact taking responsibility for their services and productions and their impact on the Planet as well as for People and their Profits.
Figures
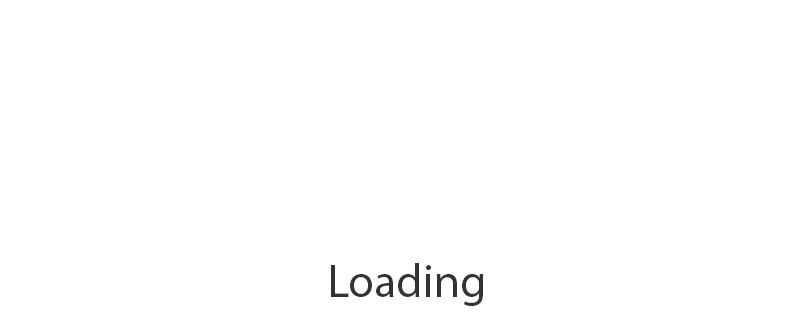
Figure 1
Depiction of the reduction of pharmaceuticals etc. in WWTPs from Castiglioni et al. (2018, p. 292, Table 2)
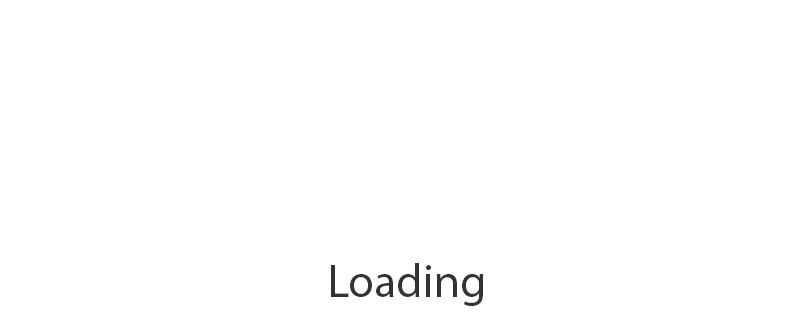
Figure 4
Vatankhah et al. (2019) – data displayed by the author for better comparison between graphs in this paper
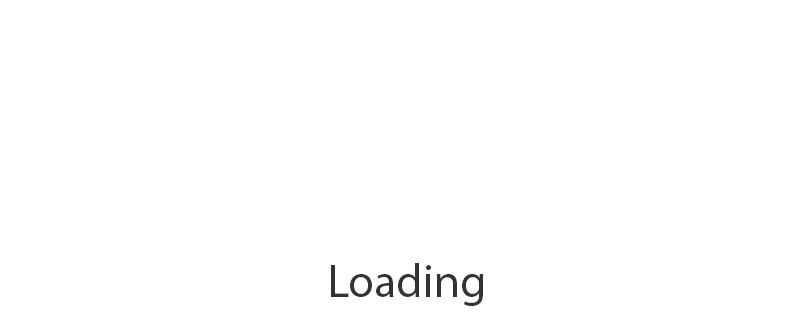
Figure 7
Cost estimates for advanced wastewater treatment technologies in 100,000 PE WWTP per m3 treated wastewater. The costs are on top of the costs for normal municipal WWTP processes, adjusted to 2020K2 in €, dst.dk, for the category: “machinery”, https://www.dst.dk/da/Statistik/emner/priser-og-forbrug/omkostningsindeks/omkostningsindeks-for-anlaeg
References
Antoniou, M.G., Hey, G., Vega, S.R., Spiliotopoulou, A., Fick, J., Tysklind, M., Jansen, J.L.C. and Andersen, H.R. (2013), “Required ozone doses for removing pharmaceuticals from wastewater effluents”, Science of the Total Environment, Vol. 456, pp. 42-49, available at: https://www.researchgate.net/profile/Henrik_Andersen/publication/236180751_Required_ozone_doses_for_removing_pharmaceuticals_from_wastewater_effluents/links/5a647a9eaca272a1581cf706/Required-ozone-doses-for-removing-pharmaceuticals-from-wastewater-effluents.pdf.
Ashfaq, M., Li, Y., Wang, Y., Chen, W., Wang, H., Chen, X. and Sun, Q. (2017), “Occurrence, fate, and mass balance of different classes of pharmaceuticals and personal care products in an anaerobic-anoxic-oxic wastewater treatment plant in Xiamen, China”, Water Research, Vol. 123, pp. 655-667, available at: https://dl.uswr.ac.ir/bitstream/Hannan/95865/1/2017%20WaterResearch%20Volume%20123%20Issue%20%20October%20%2868%29.pdf.
Blake, D.M. (1997), Bibliography of Work on Photocatalytic Removal of Hazardous Compounds from Water and Air, NREL/TP-430-22197, National Renewable Energy Laboratory, Boston, MA, Golden.
Březinova, T.D., Vymazal, J., Koželuh, M. and Kule, L. (2018), “Occurrence and removal of ibuprofen and its metabolites in full-scale constructed wetlands treating municipal wastewater”, Ecological Engineering, Vol. 120, pp. 1-5, available at: https://www.sciencedirect.com/science/article/abs/pii/S0925857418301757.
Brown, A.K. and Wong, C.S. (2018), “Distribution and fate of pharmaceuticals and their metabolite conjugates in a municipal wastewater treatment plant”, Water Research, Vol. 144, pp. 774-783, available at: https://www.researchgate.net/profile/Alistair_Brown2/publication/327180958_Distribution_and_fate_of_pharmaceuticals_and_their_metabolite_conjugates_in_a_municipal_wastewater_treatment_plant/links/5b8971b94585151fd1402c60/Distribution-and-fate-of-pharmaceuticals-and-their-metabolite-conjugates-in-a-municipal-wastewater-treatment-plant.pdf.
Capodaglio, A.G., Bojanowska-Czajka, A. and Trojanowicz, M. (2018), “Comparison of different advanced degradation processes for the removal of the pharmaceutical compounds diclofenac and carbamazepine from liquid solutions”, Environmental Science and Pollution Research, Vol. 25 No. 28, pp. 27704-27723, available at: https://link.springer.com/article/10.1007/s11356-018-1913-6.
Castiglioni, S., Davoli, E., Riva, F., Palmiotto, M., Camporini, P., Manenti, A. and Zuccato, E. (2018), “Mass balance of emerging contaminants in the water cycle of a highly urbanized and industrialized area of Italy”, Water Research, Vol. 131, pp. 287-298.
Chen, S., Blaney, L., Chen, P., Deng, S., Hopanna, M., Bao, Y. and Yu, G. (2019), “Ozonation of the 5-fluorouracil anticancer drug and its prodrug capecitabine: reaction kinetics, oxidation mechanisms, and residual toxicity”, Frontiers of Environmental Science and Engineering, Vol. 13 No. 4, p. 59.
Choi, C.H., Ko, D.H., Park, B., Choi, Y., Choi, W. and Kim, D.P. (2019), “Air-water interfacial fluidic sonolysis in superhydrophobic silicon-nanowire-embedded system for fast water treatment”, Chemical Engineering Journal, Vol. 358, pp. 1594-1600.
EPA Denmark (2018), “Environmentally friendly treatment of highly potent pharmaceuticals in hospital wastewater – Mermiss”, Report, Environmental project no. 1988, March 2018, Ministry of Environment and Food of Denmark, Environmental Protection Agency, available at: https://mst.dk/service/publikationer/publikationsarkiv/2018/mar/environmentally-friendly-treatment-of-highly-potent-pharmaceuticals-in-hospital-wastewater-mermiss/ (accessed 19 January 2021).
Fernandes, L., Lucas, M.S., Maldonado, M.I., Oller, I. and Sampaio, A. (2014), “Treatment of pulp mill wastewater by Cryptococcus Podzolicus and Solar photo-Fenton: a case study”, Chemical Engineering Journal, Vol. 245, pp. 158-165.
Fraiese, A., Naddeo, V., Uyguner-Demirel, C.S., Prado, M., Cesaro, A., Zarra, T. and Ballesteros, F. Jr (2019), “Removal of emerging contaminants in wastewater by sonolysis, photocatalysis and ozonation”, Global NEST Journal, Vol. 21, pp. 98-105.
Garcia, S., Wade, B., Bauer, C., Craig, C., Nakaoka, K. and Lorowitz, W. (2007), “The effect of wastewater treatment on antibiotic resistance in Escherichia coli and Enterococcus sp”, Water Environment Research, Vol. 79, pp. 2387-2395.
Glover, C.M., Verdugo, E.M., Trenholm, R.A. and Dickenson, E.R. (2019), “N-nitrosomorpholine in potable reuse”, Water Research, Vol. 148, pp. 306-313.
Gomes, H., Ribeiro, R., García, J., Frontistis, Z., Mantzavinos, D., Silva, A. and Faria, J. (2016), “Catalytic wet peroxide oxidation in the removal of emerging micropollutants”, in Workshop Leading Edge Technologies for the Removal of Emerging Pollutants, p. 2.
Grundfos (2016), “Full scale advanced wastewater treatment at Herlev Hospital. Treatment performance and evaluation”, Report by Grundfos BioBooster A/S, May 2016, available at: https://www.dhigroup.com/-/media/shared%20content/global/news/2016/08/evaluation%20report.pdf (accessed 24 Nov 2020).
Guo, X., Yan, Z., Zhang, Y., Kong, X., Kong, D., Shan, Z. and Wang, N. (2017), “Removal mechanisms for extremely high-level fluoroquinolone antibiotics in pharmaceutical wastewater treatment plants”, Environmental Science and Pollution Research, Vol. 24 No. 9, pp. 8769-8777, available at: https://link.springer.com/content/pdf/10.1007/s11356-017-8587-3.pdf.
Halevi, G., Moed, H. and Bar-Ilan, J. (2017), “Suitability of Google Scholar as a source of scientific information and as a source of data for scientific evaluation—review of the literature”, Journal of Informetrics, Vol. 11 No. 3, pp. 823-834.
Herrmann, J.M. (1999), “Heterogeneous photocatalysis: fundamentals and applications to the removal of various types of aqueous pollutants”, Catalysis Today, Vol. 53 No. 1, pp. 115-129.
Hofman-Caris, R., Harmsen, D. and Wols, B. (2015), “Additional UV/H2O2 treatment for removal of polar organic micropollutants at drinking water production site heel”, Report KWR 2015.082. Published Oct. 2015, available at: https://edepot.wur.nl/459288 (accessed 9 Nov 2020).
Huang, D., Hu, C., Zeng, G., Cheng, M., Xu, P., Gong, X. and Xue, W. (2017), “Combination of Fenton processes and biotreatment for wastewater treatment and soil remediation”, Science of the Total Environment, Vol. 574, pp. 1599-1610.
Janssens, R., Mandal, M.K., Dubey, K.K. and Luis, P. (2017), “Slurry photocatalytic membrane reactor technology for removal of pharmaceutical compounds from wastewater: towards cytostatic drug elimination”, Science of the Total Environment, Vol. 599, pp. 612-626, available at: https://dial.uclouvain.be/pr/boreal/object/boreal%3A185258/datastream/PDF_02/view.
Janssens, R., Cristóvão, B.M., Bronze, M.R., Crespo, J.G., Pereira, V.J. and Luis, P. (2019), “Photocatalysis using UV-A and UV-C light sources for advanced oxidation of anti-cancer drugs spiked in laboratory-grade water and synthetic urine”, Industrial and Engineering Chemistry Research, Vol. 59 No. 2, pp. 647-653.
Kaarsholm, K.M.S. (2019), “Fjernelse Af DMS Ved Behandling Med Ozon”, Report, 4th Oct. 2019, DTU Miljø Institut for Vand og Miljøteknologi, Lyngby.
Kayerød, H. and Hansen, A.A. (2019), “Interessegruppen for medicinrester i spildevand”, in Presentation (in Danish) on Spildevandsteknisk Forenings (STF) Døgnkursus, 8th Nov. 2019. Slides available from: author.
Kelbert, M., Pereira, C.S., Daronch, N.A., Cesca, K., Michels, C., de Oliveira, D. and Soares, H.M. (2020), “Laccase as an efficacious approach to remove anticancer drugs: a study OF doxorubicin degradation, kinetic parameters, and toxicity assessment”, Journal of Hazardous Materials, Vol. 409, p. 124520.
Klavarioti, M., Mantzavinos, D. and Kassinos, D. (2009), “Removal of residual pharmaceuticals from aqueous systems by advanced oxidation processes”, Environment International, Vol. 35 No. 2, pp. 402-417.
Krizman-Matasic, I., Kostanjevecki, P., Ahel, M. and Terzic, S. (2018), “Simultaneous analysis of opioid analgesics and their metabolites in municipal wastewaters and river water by liquid chromatography–tandem mass spectrometry”, Journal of Chromatography A, Vol. 1533, pp. 102-111, available at: http://fulir.irb.hr/5033/1/Krizman-Matasic_2018_OA.pdf.
Krüger (2019), “Notat. Vedr. Litteraturstudie angående fjernelse af DMS og NDMA fra vand”, Report made for Vand og Affald, Svendborg, 29.10.2019, pp. 1-10, In Danish.
Lauesen, L.M. and Bjerre, T.K. (2020), “Publicly owned company legitimacy: opportunities and challenges”, in Rendtorff, J.D. (Ed.), Handbook of Business Legitimacy: Responsibility, Ethics and Society, Springer, pp. 441-457.
Lin, H., Li, H., Chen, L., Li, L., Yin, L., Lee, H. and Yang, Z. (2018), “Mass loading and emission of thirty-seven pharmaceuticals in a typical municipal wastewater treatment plant in Hunan Province, Southern China”, Ecotoxicology and Environmental Safety, Vol. 147, pp. 530-536, available at: https://www.researchgate.net/profile/Huiju_Lin2/publication/319873320_Mass_loading_and_emission_of_thirty-seven_pharmaceuticals_in_a_typical_municipal_wastewater_treatment_plant_in_Hunan_Province_Southern_China/links/59cb248c45851556e97e3d18/Mass-loading-and-emission-of-thirty-seven-pharmaceuticals-in-a-typical-municipal-wastewater-treatment-plant-in-Hunan-Province-Southern-China.pdf.
Lindholm-Lehto, P.C., Ahkola, H.S., Knuutinen, J.S. and Herve, S.H. (2016), “Widespread occurrence and seasonal variation of pharmaceuticals in surface waters and municipal wastewater treatment plants in central Finland”, Environmental Science and Pollution Research, Vol. 23 No. 8, pp. 7985-7997, available at: https://search.proquest.com/docview/1784416964?pq-origsite=gscholar&fromopenview=true.
Márquez, J.J.R., Levchuk, I. and Sillanpää, M. (2018), “Application of catalytic wet peroxide oxidation for industrial and urban wastewater treatment: a review”, Catalysts, Vol. 8 No. 12, p. 673.
McEachran, A.D., Shea, D., Bodnar, W. and Nichols, E.G. (2016), “Pharmaceutical occurrence in groundwater and surface waters in forests land-applied with municipal wastewater”, Environmental Toxicology and Chemistry, Vol. 35 No. 4, pp. 898-905, available at: https://setac.onlinelibrary.wiley.com/doi/pdf/10.1002/etc.3216?casa_token=Js_54ao7uUMAAAAA:bHtZXJAlv093bNyQR8ndjU6ZeZQHtstRzC-HJyhzKT68kQ4g11v2CiPk9AR-2iqEGgqXs8cxB8Juhw.
Mousset, E., Wang, Z. and Lefebvre, O. (2016), “Electro-Fenton for control and removal of micropollutants–process optimization and energy efficiency”, Water Science and Technology, Vol. 74 No. 9, pp. 2068-2074.
Naturstyrelsen (2015), “Screening for humane lægemidler i vandmiljøet”, Report, Miljøministeriet, Naturstyrelsen, available at: https://naturstyrelsen.dk/publikationer/2015/feb/screening-for-humane-laegemidler-i-vandmiljoeet/ (accessed 19 January 2021) In Danish.
Nousheen, R., Batool, A., Rehman, M.S.U., Ghufran, M.A., Hayat, M.T. and Mahmood, T. (2014), “Fenton-biological coupled biochemical oxidation of mixed wastewater for color and COD reduction”, Journal of the Taiwan Institute of Chemical Engineers, Vol. 45 No. 4, pp. 1661-1665.
Oller, I., Malato, S., Sánchez-Pérez, J.A., Maldonado, M.I. and Gassó, R. (2007), “Detoxification of wastewater containing five common pesticides by solar AOPs–biological coupled system”, Catalysis Today, Vol. 129 Nos 1-2, pp. 69-78.
Pereira, C.S. (2020), “Enzymatic process as a potential treatment technology to remove anticancer drugs from wastewater: laccase-assisted degradation of etoposide”, Thesis, available at: https://repositorio.ufsc.br/handle/123456789/216321 (accessed 19 January 2021).
Plakas, K.V., Sarasidis, V.C., Patsios, S.I., Lambropoulou, D.A. and Karabelas, A.J. (2016), “Novel pilot scale continuous photocatalytic membrane reactor for removal of organic micropollutants from water”, Chemical Engineering Journal, Vol. 304, pp. 335-343, doi: 10.1016/j.cej.2016.06.075.
Ribeiro, R.S., Frontistis, Z., Mantzavinos, D., Venieri, D., Antonopoulou, M., Konstantinou, I. and Gomes, H.T. (2016), “Magnetic carbon xerogels for the catalytic wet peroxide oxidation of sulfamethoxazole in environmentally relevant water matrices”, Applied Catalysis B: Environmental, Vol. 199, pp. 170-186.
Riemenschneider, C., Al-Raggad, M., Moeder, M., Seiwert, B., Salameh, E. and Reemtsma, T. (2016), “Pharmaceuticals, their metabolites, and other polar pollutants in field-grown vegetables irrigated with treated municipal wastewater”, Journal of Agricultural and Food Chemistry, Vol. 64 No. 29, pp. 5784-5792, doi: 10.1021/acs.jafc.6b01696.
Schmidt, C.K. and Brauch, H.J. (2008), “N, N-dimethylsulfamide as precursor for N-nitrosodimethylamine (NDMA) formation upon ozonation and its fate during drinking water treatment”, Environmental Science and Technology, Vol. 42 No. 17, pp. 6340-6346, doi: 10.1021/es7030467.
Silva, D.B., Cruz-Alcalde, A., Sans, C., Giménez, J. and Esplugas, S. (2019), “Performance and kinetic modelling of photolytic and photocatalytic ozonation for enhanced micropollutants removal in municipal wastewaters”, Applied Catalysis B: Environmental, Vol. 249, pp. 211-217.
Souza, F.S. and Féris, L.A. (2016), “Hospital and municipal wastewater: identification of relevant pharmaceutical compounds”, Water Environment Research, Vol. 88 No. 9, pp. 871-877, available at: https://onlinelibrary.wiley.com/doi/pdf/10.2175/106143016X14609975747603?casa_token=ARNZzZb4HHMAAAAA:pWp6sXFSyyeKbtPDlo58YDaF2lgyPsSSIsLNLz9HvBttWQAzEPDiA7YiaMNOZGnclYB-dvXJwrZ0-Q.
Spataro, F., Ademollo, N., Pescatore, T., Rauseo, J. and Patrolecco, L. (2019), “Antibiotic residues and endocrine disrupting compounds in municipal wastewater treatment plants in Rome, Italy”, Microchemical Journal, Vol. 148, pp. 634-642, available at: https://www.sciencedirect.com/science/article/abs/pii/S0026265X19303315.
Tang, K. (2018), “Removal of pharmaceuticals from hospital wastewater by staged biofilm and ozone polishing”, PhD Thesis, Technical University of Denmark, DTU, Kgs. Lyngby.
Tang, K., Ooi, G.T., Torresi, E., Kaarsholm, K.M., Hambly, A., Sundmark, K., Lindholst, S., Sund, C., Kragelund, C., Christensson, M., Bester, K. and Andersen, H.R. (2020a), “Municipal wastewater treatment targeting pharmaceuticals by a pilot-scale hybrid attached biofilm and activated sludge system (Hybas™)”, Chemosphere, Vol. 259, 127397, available at: https://www.sciencedirect.com/science/article/abs/pii/S0045653520315915.
Tang, K., Rosborg, P., Rasmussen, E.S., Hambly, A., Madsen, M., Jensen, N.M., Hansen, A.A., Sund, C., Andersen, H.G., Torresi, E., Kragelund, C. and Andersen, H.R. (2020b), “Impact of intermittent feeding on polishing of micropollutants by moving bed biofilm reactors (MBBR)”, Journal of Hazardous Materials, Vol. 403, 123536.
Tang, K., Ooi, G.T., Spiliotopoulou, A., Kaarsholm, K., Sundmark, K., Florian, B., Kragelund, C., Bester, K. and Andersen, H.R. (2020c), “Removal of pharmaceuticals, toxicity and natural fluorescence by ozonation in biologically pre-treated municipal wastewater, in comparison to subsequent polishing biofilm reactors”, Water, Vol. 12 No. 4, p. 1059.
Thiebault, T., Boussafir, M. and Le Milbeau, C. (2017), “Occurrence and removal efficiency of pharmaceuticals in an urban wastewater treatment plant: mass balance, fate and consumption assessment”, Journal of Environmental Chemical Engineering, Vol. 5 No. 3, pp. 2894-2902, available at: https://hal-insu.archives-ouvertes.fr/insu-01531858/file/Manuscriptedited.pdf.
Thuy, H.T.T. and Nguyen, T.D. (2013), “The potential environmental risks of pharmaceuticals in Vietnamese aquatic systems: case study of antibiotics and synthetic hormones”, Environmental Science and Pollution Research, Vol. 20, pp. 8132-8140.
Vatankhah, H., Riley, S.M., Murray, C., Quiñones, O., Steirer, K.X., Dickenson, E.R. and Bellona, C. (2019), “Simultaneous ozone and granular activated carbon for advanced treatment of micropollutants in municipal wastewater effluent”, Chemosphere, Vol. 234, pp. 845-854, available at: https://www.researchgate.net/profile/Hooman_Vatankhah/publication/333809994_Simultaneous_ozone_and_granular_activated_carbon_for_advanced_treatment_of_micropollutants_in_municipal_wastewater_effluent/links/5ddf0e5b92851c83644e355d/Simultaneous-ozone-and-granular-activated-carbon-for-advanced-treatment-of-micropollutants-in-municipal-wastewater-effluent.pdf.
Verma, P. and Samanta, S.K. (2018), “Microwave-enhanced advanced oxidation processes for the degradation of dyes in water”, Environmental Chemistry Letters, Vol. 16 No. 3, pp. 969-1007.
Wang, W.L., Wu, Q.Y., Huang, N., Xu, Z.B., Lee, M.Y. and Hu, H.Y. (2018), “Potential risks from UV/H2O2 oxidation and UV photocatalysis: a review of toxic, assimilable, and sensory-unpleasant transformation products”, Water Research, Vol. 141, pp. 109-125.
Xiong, J.Q., Kurade, M.B. and Jeon, B.H. (2018), “Can microalgae remove pharmaceutical contaminants from water?”, Trends in Biotechnology, Vol. 36 No. 1, pp. 30-44, available at: https://d1wqtxts1xzle7.cloudfront.net/60275140/Can_Microalgae_Remove_Pharmaceutical_contaminants_from_water20190812-69293-w99hke.pdf?1565663342=&response-content-disposition=inline%3B+filename%3DCan_Microalgae_Remove_Pharmaceutical_Con.pdf&Expires=1598863951&Signature=aVBVGIEmZKhtOaurVY0WjxCmd8sxib44PF9f4x9AqOewWi∼nsivQIQqUa8Vq7NFtQ44mJiUeKWmPdgTe1QYm1E4pZCAELKtQiyZPrbpk84XU3KoMv5mgjfcNqOerxtsrb4IHs1iEFHrEpQTCcD98Q5MYm3S5n0BMrlcqDoY2q4E0Q7HXXVbzUCz1ra50aub6vmc7ftOlflgIFs4mjQJDVEMbmhffzh0r2thbwZ-OiA9vcU∼TDD∼OJNkLz∼ed3Lxac2uWgB8wWaSY3DAHTjJgmFlHoLaXTULoLOGUmNwF8xzAx-1ziJ6lxXjwhf8Zff8SYgOkf3R-WDwQbdZiEOj7cA__&Key-Pair-Id=APKAJLOHF5GGSLRBV4ZA.
Corresponding author
About the author
Linne Marie Lauesen is an engineer and has a PhD in Corporate Social Responsibility from Copenhagen Business School with special focus on the water sector. Beyond this, Linne has made a post doc study in Corporate Social Responsibility with special focus on the food sector in Denmark, also from Copenhagen Business School. Linne works today as an industrial researcher based in a water and waste utility company (Vand og Affald/Water & Waste Denmark) based in her hometown Svendborg, Denmark. From here, she is engaged in both national and international research projects relevant for the water, waste water and waste sectors. Linne has furthermore specialized in Life Cycle Assessments, which she uses in her research and daily work as a project manager. Linne has a strong publishing portfolio related to environmental research and technologies in the above sectors.