Abstract
Purpose
Blockchain technology is provoking significant disruptions, thereby affecting supply chain management. This study endeavoured to advance research regarding blockchain-based supply chain traceability by identifying the opportunities and limitations that accompany the adoption of public blockchains. Therefore, the purpose of the study is to contribute to contemporary supply chain research by an assessment of blockchain technology and its linkages to traceability.
Design/methodology/approach
This paper is conceptual. The authors summarised the relevant literature on the concepts of supply chain traceability, conceptualised key elements exclusive to the public blockchain and highlighted opportunities and limitations in implementing traceability using blockchains.
Findings
Incompatibilities were identified between general traceability and the public blockchain. However, when embracing the blockchain's privacy model, the blockchains can support information exchange in supply chains where vulnerability towards third parties, the confidentiality of information, or the privacy of participants are concerns. Furthermore, the public blockchain can support areas of supply chains where institutional interest is lacking.
Originality/value
This is one of the first papers in an international supply chain management journal to critically analyse the intersection of specific blockchain characteristics and supply chain traceability requirements. The authors thereby add to the discussion of designs for a disintermediated, peer-to-peer models and guide researchers and practitioners alike in exploring the application of disruptive change from blockchain technologies. By setting focus on the privacy model, the paper identifies the potential application and future research approaches to exploit the elementary strength of the blockchain.
Keywords
Citation
Bischoff, O. and Seuring, S. (2021), "Opportunities and limitations of public blockchain-based supply chain traceability", Modern Supply Chain Research and Applications, Vol. 3 No. 3, pp. 226-243. https://doi.org/10.1108/MSCRA-07-2021-0014
Publisher
:Emerald Publishing Limited
Copyright © 2021, Oliver Bischoff and Stefan Seuring
License
Published in Modern Supply Chain Research and Applications. Published by Emerald Publishing Limited. This article is published under the Creative Commons Attribution (CC BY 4.0) license. Anyone may reproduce, distribute, translate and create derivative works of this article (for both commercial and non-commercial purposes), subject to full attribution to the original publication and authors. The full terms of this license may be seen at http://creativecommons.org/licences/by/4.0/legalcode
1. Introduction
Poor information sharing has been identified as an important company-side supply chain risk. Distributed networks can improve the availability of information and systems providing accurate and timely traceability information are suitable to reduce supply chain risks (Wang et al., 2020). Producers and customers alike demand verifiable evidence of traceability as an important criterion for product sustainability. In this respect, tracking data can aid the demonstration of regulatory, social and environmental compliance. Stringent legal provisions and regulations, as well as the high costs of product recalls, strongly motivate traceability as a feature of contemporary supply chain management (Kshetri, 2018). Furthermore, capturing the history of a product through a supply chain enables the optimisation of processes and the prediction of downstream time and quality issues, thereby engendering advantages related to costs, time and customer satisfaction (Hald and Kinra, 2019). Traceability can also accelerate innovation and sustainability within an organisation, leading to competitive advantage through differentiation (Bosona and Gebresenbet, 2013).
The blockchain is set to accelerate digital supply chain integration (Korpela et al., 2017) and is certain to revolutionise supply chain management' (Manupati et al., 2019, p. 16) as well as the transportation and logistics industry (Koh et al., 2020). With its effectiveness in advancing peer-to-peer transactions in privacy without third-party intervention, a blockchain-based solution can mitigate risks associated with data manipulation, compromised privacy, or compliance with government rules and regulations (Min, 2019), while reducing auditing costs (Choi et al., 2020). Several researchers have identified supply chain management as an area that can benefit from blockchain implementation by achieving radical transparency (Voshmgir, 2017), sighting revolutionary change to supply chain traceability (Abeyratne and Monfared, 2016). Accordingly, blockchains have been increasingly implemented in areas with increased traceability requirements such as the food, fashion and logistics industry, thus emphasising the relevance of this topic.
Examples of blockchain adoption in supply chain management are as follows: FarmaTrust (www.farmatrust.com) provides blockchain and AI-based systems for the pharmaceutical and healthcare sector, and IBM Food Trust (www.ibm.com/blockchain) is a blockchain-based platform that connects all food industry players to shared records of food system data. Namahe (namahe.io) is a fashion value chain-orientated blockchain that focuses on social responsibility, and Provenance (www.provenance.org) is a platform empowered by blockchain innovations in the collection and sharing of key product information. Tracr and De Beers (www.tracr.com and www.debeers.com, respectively) developed a platform that securely tracks a diamond across an entire value chain. Tradelens (www.tradelens.com) is a digital platform that empowers businesses and authorities along a supply chain with a single, secure source of shipping data. Despite these developments, however, these projects are largely centred on capturing the advantages of blockchain technologies, failing to engage with the institutional innovation potential of blockchains (Blossey et al., 2019).
Additionally, an estimated 90% of blockchain-based business projects were initiated only after 2015 (Behnke and Janssen, 2019). Yaga et al. (2018) identified that, while there is a lack of knowledge about the technology, “there is a tendency to want to apply it to every sector in every way imaginable” (Yaga et al., 2018, p. 4). Supporting this contention, Australia's Digital Transformation Agency (2019) concluded that “for many uses of blockchain, there are currently other mature technologies that may be more suitable for immediate use”. While there is plenty of discussion regarding the advantages and disadvantages of blockchain technologies, a gap exists with regards to the blockchains' proficiency to effectively match supply chain traceability characteristics (Cole et al., 2019, p. 480). With consideration for the above-mentioned issues, the current research analysed the tension between supply chain traceability needs and blockchain capabilities. We base our study on general supply chain traceability requirements without limiting the research to specific stakeholders or industries. On the blockchain side, we focus on the disruptive decentralisation and disintermediation characteristics and analyse the consequences for governance in distributed, anonymous configurations. Based on the identified key elements, we conducted a conceptual examination guided by the following research questions (RQs):
Which compatibilities and conflicts between the blockchain technology and supply chain traceability can be identified?
How can a public blockchain significantly affect supply chain traceability?
In this line, our research adds to the discussion of alternative designs for a disintermediated pure peer-to-peer model, such as public or private and permissioned or permissionless blockchains (Narayanan et al., 2016). In these permissioned blockchains, trust issues are affected because the “administrators of those systems act as an administrator of trust by granting users admission and permissions” (Yaga et al., 2018). Moving the blockchain into a private or permissioned environment remove anonymity and install trust between the participants, rendering blockchain's trust mechanism redundant and “defeats the purpose of considering a blockchain solution to begin with” (Garrard and Fielke, 2020, p. 3). Our conceptualisation shows that selecting a suitable blockchain innovation individually to address supply issues (Bai and Sarkis, 2020) might cast doubt on the overall benefit of the blockchains in question. Several researchers raised the concern that this “pick-and-choose” attitude towards blockchain innovations might project these adoptions as being little more than the “new database tool” rather than the “next Internet” (Tatar, 2019). Consequently, private blockchains would only be a confusing name for shared databases (Narayanan, 2015). Therefore, we focus this research on public blockchain in the context of supply chain traceability. Our findings hence guide researchers and practitioners alike in explorations that centre on either incremental innovative application or disruptive change from blockchain technologies and offer research directions for blockchains in supply chain management, focussing on governance and anonymity.
The remainder of this paper is structured as follows. We first present key concepts of blockchain and supply chain traceability as well as introduce associated fundamental concepts. We then present our analysis, discussing the findings from a traceability and blockchain perspective. Implications for researchers and managers are identified. Finally, limitations and opportunities for further research are provided.
2. Literature background
2.1 Supply chain traceability
Supply chain traceability is the capturing and analysis of custody, transformational and environmental information of material flows through the supply chain aiming to satisfy stakeholder needs regarding environmental, social and economic performance; compliance; and quality (Jansen-Vullers et al., 2003; Skilton and Robinson, 2009; Kshetri, 2018). Industries are confronted with specific issues and stakeholder pressures, Hastig and Sodhi (2020) set the resulting business requirements into context with potential effects of the blockchain (and other technologies) and critical success factors for traceability-system implementation. They do not look at typical operationalisations of traceability, which is the specific issue addressed in this paper.
Traceability typically consists of gaining insights retroactively (though it may also serve to anticipate events, discussed below), serves as an important component of the business information system and can be a source of competitive advantage for businesses as it allows them to ensure a transparent and sustainable supply chain (Garcia-Torres et al., 2019). Tracking, on the other hand, is the determination of the current information that indicates the status—like the location—of an object being tracked downstream through the supply chain (Fritz and Schiefer, 2009). Tracking, also referred to as forward traceability, occurs in real-time (Behnke and Janssen, 2019) and is “the ability to follow the downstream path of a product along the supply chain, possibly according to some specific criteria” (Bechini et al., 2008, p. 343).
With an efficient and effective system transmitting accurate, timely, complete and consistent traceability information, a supply chain can significantly reduce its operation costs, increase productivity and improve customer confidence (Kshetri, 2018; Bailey et al., 2016). To achieve this, companies need to cooperate to determine and fulfil the requirements for traceability so that all information necessary to the supply chain can be managed efficiently and effectively (van Dorp, 2002). Certifications and related documents are major means of validating product origins and sustainability fulfilments, for example (Bailey et al., 2016), for customers who are demanding more transparency from brands, manufacturers and producers throughout the supply chain about the origin of products as an expression of their concern for sustainability. Research on the subject has clearly stated that “traceability is required to recall what has already occurred in time and that “it must be possible to trace from one entity to another” (Kim et al., 1995, p. 109).
Four principles of traceability (Table 1) have been identified as (1) the unique identification of products, logistic units and locations; (2) traceability data capture and recording; (3) management and traceability data retrieval; and (4) traceability data communication (Global Standards One, 2017; Storøy et al., 2008). A unique identification of products as well as the links between components, combined in internal processing, are required (Jansen-Vullers et al., 2003). To enable traceability, products, including materials, components and assemblies, must be uniquely identifiable so that at each stage of the supply chain, the physical location of a specific object can be determined. This facilitates logistical activities, recalls and the provision of information to consumers and other stakeholders (Moe, 1998; Opara and Mazaud, 2001).
Moe (1998) takes the concept a step further by making a distinction between chain traceability and internal traceability. Chain traceability focuses only on a subset of information, collecting data from the movement of an object through the supply chain from the source to the end customer. Internal traceability, on the other hand, links objects to more granular traceability information collected from the transformation process generated by partners within the supply chain. Identifying objects and their transformation relationships (in the form of splits or joins) is the key to product traceability (Storøy et al., 2008). Capturing the history of a product through the supply chain enables the optimisation of processes as well as the demonstration of regulatory compliance (Hald and Kinra, 2019; Kolk, 2008). While Kim et al. (1995) view traceability as occurring “backwards in time”, other authors recognise the importance of tracking data for predicting downstream events along the supply chain. Tracking data enables prediction and early communication of defects and adverse events (Skilton and Robinson, 2009), quality (Bollen, 2005) or arrival time (Biagioni et al., 2011).
The traceability system refers to all methods, procedures and routines used to manage traceability in a supply chain. Traceability systems are used by individual partners to increase visibility across their own organisations and contribute to end-to-end supply chain traceability as well (Global Standards One, 2017).
2.2 Achieving supply chain traceability through information technology and related challenges
Traceable information includes supply chain trading volumes, address information like the origin and source of a product, as well as dimension or chemical composition (Bosona et al., 2018), all of which can help to validate sustainable and ethical supply chain compliance (Pournader et al., 2020). However, smaller scale companies often use manual, paper-based traceability, even though it limits the ability to accurately record, store and query data to identify and track products. To these companies, such pencil-and-paper systems represent a low-cost alternative to sophisticated information technology-based solutions (Karippacheril et al., 2017). The following requirements need to be fulfilled in order to achieve technology-driven supply chain traceability:
Data, generated continuously by multiple entities, must be resilient against tampering or falsification. Integrity and reliability of the information must also be ensured. Handling the large amount of data generated along the supply chain in an efficient, secure and economic way is, therefore, a key challenge of traceability (Qiao et al., 2018).
Continuous monitoring of conditions such as temperature, humidity and vibration can be applied to assess supply chain quality. All of this information can be analysed and used to either minimise quality loss or improve upon transportation services (Chaudhuri et al., 2018)
Because systems are required to operate in complex supply chains with large, dynamic groups of participants, the cost can be high (Aung and Chang, 2014). Accordingly, the accuracy, storage and timely retrieval of such high volumes of traceability data present challenges. Additionally, the need to ensure the privacy, security and reliability of tracking data limits the effectiveness of supply chain traceability systems.
While the introduction of information technology to ensure comprehensive product traceability throughout the supply chain does enable companies to control cost and meet privacy, security, reliability and other legal requirements (Aung and Chang, 2014), when investigating the blockchain (discussed in the following section), Behnke and Janssen (2019) found that the standardisation of processes and master data using information technology, in general, is of critical concern for traceability. Bechini et al. (2008) concluded that a centralised approach to traceability systems is neither realistic nor efficient. Therefore, a decentralised, rather than centralised, storage of data generated in similarly decentralised process steps in the supply chain is necessary to realise traceability in the context of the digitisation of supply chain activities. Figure 1 shows the different models that can be used to organise traceability data. In the centralised model, all parties share their information in a central repository. The central database holds the complete set of its systematically enhanced traceability data at one point. However, access rights might be set individually for all parties. The central node might therefore become a bottleneck for communication and processing (Rao et al., 1993). In the networked model, the participating parties keep their individual data in local systems, providing supply chain partners with query access to relevant information. In the cumulative model, traceability data are enhanced as they move together with the material downstream along the supply chain from one partner to the next. Here, data are only shared with downstream partners. In the decentralised model, as used in the blockchain, all data are systematically enhanced and shared with all supply chain partners. Elimination of central processing and maintenance of a local copy of the complete set of traceability data by each partner ensures high resilience (Rao and Durrant-Whyte, 1991). While centralised and decentralised models both provide a single traceability dataset, the cumulative scenario provides only local and upstream information from the individual dataset. To achieve traceability in the networked scenario, multiple distributed datasets of the various partners need to be queried (Global Standards One, 2017).
3. The blockchain
The blockchain, the basis of the bitcoin network, is a decentralised, distributed ledger “for electronic transactions without relying on trust” (Nakamoto, 2008, p. 8). The application functions as an immutable ledger that allows for transactions to occur directly between anonymous parties in a decentralised fashion (Zheng et al., 2017). The information is stored transparently, chronologically and securely (Abeyratne and Monfared, 2016). While blockchain ensures persistent storage and consistency of public data, the technology does not provide interfaces for retrieval, update, evaluation or management of users, data structure or the data itself. In summary, the blockchain is characterised by disintermediation, decentralisation, transparency and immutability (Rodrigo et al., 2018; Wang et al., 2019a) (see Table 2).
Nonetheless, certain database functionalities similar to those offered by blockchain, such as the management of large amounts of shared data, a coherent database state, protection against misuse, data recovery, security control for accessing and/or changing information, dataset distribution and transparent access has been in place for decades (Abiteboul et al., 1996). Furthermore, smart contracts (Szabo, 1994), hash trees (Merkle, 1979), digital timestamping (Haber and Stornetta, 1991) and cryptography (Diffie and Hellman, 1976) have also been part of the discussion surrounding data management since the second half of the last century.
The disruptive characteristics of the blockchain result, rather, from its ability to break up organisational governance structures and established business models (Fridgen et al., 2017; Voshmgir, 2017). By replacing reliance on centralised architectures and trusted third parties with blockchain's decentralised privacy model, the technology introduces a serious disruptive potential for traditional business processes (Casino et al., 2019). Blockchain's disruptive decentralisation and disintermediation characteristics can therefore impact supply chain management (Queiroz et al., 2019).
3.1 Privacy model - disintermediation
Blockchain's disintermediation is reflected in its new privacy model. Nakamoto (2008) developed this privacy model, wherein transactions are public while the identities of the transacting parties remain protected (Figure 2). The traditional mediator is replaced by governing mechanisms, allowing participants to reach a consensus on transaction validity. In this model, all participants are equal, and all transactions are visible to and verifiable by the public. Privacy is achieved by protecting transacting parties through the anonymity enabled by public-key cryptography. However, to validate the public transactions of anonymous nodes, a new validation process had to be developed (Nakamoto, 2008). Due to the lack of a central governing party, a permissionless consensus mechanism allows nodes to coordinate the system status by mass collaboration (Bogart and Rice, 2015). Consistency between the different participants is ensured through this protocol.
In fact, when considering fully or partially replacing traditional systems with technological alternatives, the discussion is ultimately about the redistribution of power from well-established legal, social and financial institutions (Narayanan et al., 2016, p. 303), introducing risks of conflicts with existing laws and regulations (DuPont, 2018).
3.2 Decentralisation
All participants are equal nodes in a pure peer-to-peer decentralised and distributed network with “no central authority” (Nakamoto, 2008), whereby “any single, arbitrarily chosen terminal entity can be removed from the network without having the network suffering any loss of network service” (Schollmeier, 2002, p. 102). The decentralised architecture leads to increased resilience of the system, as it removes any single point of failure. However, decentralisation requires autonomous participants to provide resources for transmitting, storing and validating the datasets. Furthermore, the requirements present challenges to the participation of internet-of-things devices in private or public blockchains (Lao et al., 2020).
While all participants have fast read and write access to their locally-stored copies of the data, access to new, validated transactions is delayed. Block formation, block validation and the propagation of new blocks, through the decentralised network, introduce delays in the release of transactions (Sompolinsky and Zohar, 2013).
3.3 Transparency
The Blockchain enables “transparency among untrusted stakeholders” (Scully and Hobig, 2019) by protecting them from fraudulent transactions (Nakamoto, 2008). While transparency is created through information sharing and can eliminate information asymmetries, this falls alone short of the radical transparency (Voshmgir, 2017) promoted by the blockchain. All transactions are visible to all participants. Everybody can view and verify the system's transactions. As transactions in the new privacy model are exposed to the public, the identity of the transacting participants is protected. Blockchain realises this anonymity using changing pseudonyms based on public-key cryptography (Diffie and Hellman, 1976) and the unlinkability of participants and objects. Due to their anonymity, there can be no trust relationship established between the nodes. Changing the public key address for each transaction prevents transactions from being linked to a participant. Additionally, the blockchain does not provide linkage to off-system assets. The blockchain does not connect the transaction to real-world objects, as this could break anonymity (Wang et al., 2019a).
3.4 Immutability
Blocks of transactions are created when the blocks are timestamped, linked through a hash to the previously created block (Merkle, 1979) and signed (Haber and Stornetta, 1991). The transaction is “append only” and protected against reversal. Network participants can audit the integrity and authenticity of a transaction by recalculating the hash value of each block and comparing it to the stored value (Tapscott and Tapscott, 2016). Nakamoto (2008) explains this protection afforded to the transaction as follows: “to modify a past block, an attacker would have to redo the proof-of-work of the block and all blocks after it and then catch up with and surpass the work of the honest nodes” (Nakamoto, 2008). Consequently, the chain becomes tamper-resistant, as manipulations of transactions its data can be detected and rejected by the network.
4. Conceptual analysis
We will now analyse traceability requirements and blockchain capabilities from both viewpoints. First, by looking at blockchain from the perspective of traceability, we will analyse how the key elements of traceability are supported by the characteristics of blockchain. Second, by looking at traceability from a blockchain perspective, we will analyse the impact that the adoption of blockchain concepts would have on traceability.
4.1 Traceability perspective on the blockchain
Supply chain traceability relies to a large degree on uniquely identifiable objects and actors. Linking objects, processes and locations on both chain and internal levels is a fundamental requirement. The blockchain relies on individuals' anonymity to allow the transactions to be visible and verifiable for all participants.
To enable meaningful capture and recording of traceability data, standardisation of processes and data is required. While the blockchain does not provide this standardisation, the distributed nature of data storage provides availability and resilience. Data are written and read by the local instance of the data store and distributed throughout the network. However, distribution and verification introduce latency mostly driven by data and network size. The quality of the captured data has to be high, as later modification of accepted data is not allowed.
Linking process and data is a key requirement for supply chain traceability. While the visibility of all transactions in the blockchain appears to enable the connection between internal and chain transactions, the linkage presents a threat to the required anonymity. On the blockchain not only are individuals' pseudonyms unlinkable, but the transferred on-system electronic assets are also not interlinked.
As the communication of data between the distributed data stores is anchored in the blockchains' design, and reporting can be performed against local instances of the data, its availability is limited by the speed of propagation. In other words, the latency limits the ability to report the current state. Table 3 summarises these findings.
4.2 Blockchain's perspective on traceability
Now, we revert the perspective and analyse traceability from a blockchain perspective. Disintermediation is a key aspect of the blockchain privacy model, in which the consensus mechanism replaces the mediator. Producers, focal firms and governments are key stakeholders in supply chain traceability with an interest to govern the system and the ability to provide resources and processes to fulfil the role of the mediator. Furthermore, many proposed traceability systems are envisaged as closed groups of users or permissioned access rights, introducing an administrative authority. The presence of centralised mediating authorities, therefore, impacts anonymity in the blockchain.
In the absence of a central authority in the blockchain, identities are protected and transactions openly visible and verifiable. Therefore, transparency is dependent on the concept of anonymous identities to preserve overall privacy. Data transparency, under the blockchains' privacy model, supports the need of supply chain traceability. However, provenance and custody information are key interests of supply chain traceability, and blockchains' anonymity requirement restricts the possible linkages.
The decentralisation of blockchains mirrors in many aspects the governance of supply chains. The fully decentralised approach, necessitated by the absence of a central third party, is at the core of the blockchain. However, supply chains, with heterogeneous capabilities and interests of supply chain partners, present challenges for the decentralisation of data. Specifically, companies' financial and technical resources to distribute, store, validate, analyse, or otherwise exploit large amounts of traceability data might differ greatly. Additionally, while supply chain traceability requires constant participation of all partners in the supply chain, the blockchains' pure peer-to-peer approach allows participants to join or withdraw from the network without impacting the blockchain. Furthermore, latency in the blockchain is introduced through block building, validation and network distribution. This latency hinders blockchain adoption when supply chain traceability requires real-time information.
The blockchain's approach to chaining and validating data records strengthens the immutability of information. This supports the demand of supply chain management for trustworthy, immutable information. However, the correction of erroneous data and removal or archiving of expired data from fast-growing traceability data pools are limited. The findings of this blockchain perspective of traceability are summarised in Table 4.
4.3 Joining both perspectives
In the blockchain, validation of transactions through all participants is enabled by the data transparency of stored transactions, matching the need for transparency of product data in supply chain traceability. Therefore, companies are sharing all information with the whole supply chain or even with the public. Information like production volumes and supplier and client relationships can be a source of competitive advantage and sharing might weaken their position when negotiating contracts with suppliers or when competing within the market. Therefore, restrictions might be applied when capturing information of product, service or financial flows between participants. Confidentiality of information can only be protected by maintaining the anonymity of the sharing supply chain partner. However, the flow of identifiable products, in contrast to the value of a currency, will enable the deciphering of the partner's identity.
The peer-to-peer network with its equally privileged, equipotent participants and distributed architecture are at the base of the technology. Disintermediation as a key concept removes exposure to any centralised governance or control. However, producers or retailers, governing access to the traceability system and validating content, often drive traceability projects. The power to force adoption of traceability systems, as well as the ability to benefit directly from their implementation, resides with focal firms rather than the collective of supply chain partners. The administration of access to the system as a whole and specific function within the system requires some form of intermediary to govern access rights. To be validated, partners will have to give up their anonymity. Anonymity is further threatened by a need to apply unique identifiers when linking internal processes and data to supply chain partners for traceability.
Due to the lack of a central organisation, all participants will store all data and communicate directly with each other. Access to the data is ensured, as validated copies are kept locally, and transactions are being executed between the two partners directly. Therefore, data availability is high, and reporting on the traceability information can be performed efficiently. However, distribution and validation in the background will cause delays, and verified information will not immediately be available to up- or downstream partners for reporting. The process of continuously synchronising all system transactions across the network puts the onus on every participant to communicate, store and validate all system data and constrains transaction frequency and size. Additionally, not every supply chain partner might be able to benefit at all from the communicated data. While in the blockchain partners are free to join or withdraw from the network, traceability expects all partners to share required transactional information without delay.
Once the data in the blockchain are validated, they cannot be changed. This immutability secures the reliability of the information stored in the system. However, tamper resistance limits the ability to correct data errors. Therefore, the immutability of the blockchain is of greatest benefit when systems capture and record data in an automatic, paperless way. As the complete chain of transactions is required to validate block data, the ability to remove historic transactions is impaired. Consequently, an ever-growing blockchain will stress the resource requirements of all participants in the supply chain.
Summarising the joint perspectives, we propose a public blockchain-based supply chain traceability framework (Figure 3), highlighting the interlinked key concepts.
Disintermediation in the peer-to-peer network, at the core of the blockchain, is not a key requirement for traceability. While transparency is present in the blockchain and required by traceability, confidentiality relies on anonymity. However, anonymity cannot be maintained when observing flows of objects in a closed group of participants. Decentralisation causes latency and increased resource consumption due to the duplications in governance, storage, data processing and network transfer. Therefore, blockchain can play a role in traceability when (1) no intermediary is present or wanted, (2) confidentiality is not required or beneficial information can be recorded anonymously and (3) transactions where error-rate, size and velocity are low or not of high relevance.
5. Discussion
In essence, the disruptive privacy model innovation of the blockchain will not come to fruition in general supply chain traceability. Potential intermediaries, system owners and administrators are present and anonymity in many cases undesired. However, some aspects of technologies adopted by the blockchain can improve traceability implementation.
Supply chain experts view the confidentiality of information as important to their competitive advantage (Wang et al., 2019b) and may not be willing to share critical information due to privacy and security concerns (Huang et al., 2003). The blockchain enforces privacy of the transparently stored information through participant anonymity. Supply chain performance might benefit from shared transactional information, even if the identities of the participants remain unknown.
The design of blockchains leads often to inefficient use of infrastructure (Babich and Hilary, 2020). Transactional requirements determine latency in the system and storage requirements (Sompolinsky and Zohar, 2013). The blockchain has limited storage capability as it is required to maintain the full history of all blocks and transactions across all participants of the blockchain network (Lu et al., 2018). While the actual transaction records can be removed at some point (Nakamoto, 2008), the blockchain will continuously grow. These limitations to scalability will inhibit the size of the blockchain implementation (Chang et al., 2020). Improvements to storage and computing, and the removal of intermediaries in the flow of information can, potentially, improve the speed of information flow (Saberi et al., 2019). Therefore, technological feasibility is one of the critical success factors (Hastig and Sodhi, 2020) and these infrastructure implications need to be recognised when evaluating blockchain as a design option. The lack of practical application examples, low adaptation rate might well be the result of a lack of operational suitability.
Supply chains may require user-specific type of information disclosure (Choi et al., 2020), limiting the number of participants (Saberi et al., 2019; Chang et al., 2020) and needs for unique object traceability weaken immutability and privacy in the blockchain (Zheng et al., 2017). Changes to the privacy model through centralisation and linkages of transactions or identities carry the risk of systematic de-anonymization (DuPont, 2018).
Disruptions from the blockchain are expected to deliver benefits in supply chain management (Queiroz et al., 2019). The blockchain disruptive force is rooted in eliminating intermediaries, allowing peers to transact directly in privacy and removing vulnerability to a controlling party. Furthermore, direct transacting with unknown, and therefore untrustworthy, suppliers could be enabled through the blockchain's governance model. The result could be the elimination of mediators, enabling a shorter, less costly supply chain. Research by Wang et al. (2019b) suggests that even if the adoption of the blockchain leads to disintermediation in the short term, the traditional intermediaries might be replaced by new intermediaries over time, reversing at least some blockchain benefits. Therefore, the sustainability of a disintermediated traceability system, free of any central authority, should be investigated early.
Disintermediation is the driver of potentially disruptive change from the blockchain. In the tension between disintermediation and business case lies an opportunity for researchers, not bound by the need to develop a business case. Advancement of the privacy model, rather than focussing on technical aspects, could prove to be highly beneficial for specific traceability challenges as well as overall supply chain performance. Supply chain spheres, where institutional interest is lacking, supply chain partners struggle to coordinate their activities, or individual infrastructure investment cannot be justified, could be rewarding areas of research. Specifically, challenges in the sharing of confidential information could be addressed by blockchain's privacy model. The question, if the new privacy models could elevate supply chain information needs effectively, can be a rewarding area of future research. Additionally, supply chain performance could be improved in areas where costly intermediaries are being replaced by approaches embracing blockchain's disintermediation and thereby potentially shortening supply chains. The adoption of pure peer-to-peer information sharing has the power to impact supply chain design and cost significantly. The role of the blockchain might not be one of the central supply chain traceability systems, but rather a supporting extension (van Hoek, 2019). More research is needed in this direction.
Strong links have been found between information sharing technology and performance (Prajogo and Olhager, 2012). Benefits found, when investigating blockchain cases, could arise from overall technology adoption (Garrard and Fielke, 2020). Case study research, investigating blockchain application in productive use, could shine light on the source of blockchain's disruptive force in supply chain management. Research should identify the impact achieved through blockchain adoption and where this impact is rooted within the systems' technology. This benefit needs to be set into context to potential alternative approaches applying other mature technologies like shared database systems.
5.1 Limitations
In this study, we did not distinguish between the different types of supply chains, product types, or stakeholders. Traceability privacy requirements, for example, the confidentiality needs of sub-suppliers, or the number of intermediaries involved, might differ. Furthermore, we did not dissect the technological fabric of the blockchain and engage with these single technological components.
6. Conclusions
Based on a systematic conceptual analysis of the blockchain and supply chain traceability, the study identified several incompatibilities between the characteristics of the blockchain and general traceability requirements. Not much of the revolution is left once the blockchain is wrapped into an, ultimately purpose-defeating, permissioned, distributed system, to overcome the public blockchain's limitations. The disruption of established business models in supply chains is unlikely to originate in the incremental enhancement of shared database functionalities. The blockchain is woven out of well-recognised technologies of the last century and boasts unique characteristics that allow for disruptive innovation. Blockchain-washing based in inspiration from and appreciation of blockchain characteristics obscures the view on potentially disruptive blockchain developments. We can adopt the single technologies and enhance information sharing in supply chains, or elsewhere. However, the true force of the blockchain can only be realised by the adoption of the privacy model.
By leveraging the framework that we extracted from our analysis (Figure 3), practitioners can at an early stage identify opportunities from the blockchain for their traceability challenges and validate their business requirements towards supply chain traceability against the interdependencies of public blockchain design characteristics. Furthermore, from a managerial point of view, it will be possible to evaluate the potential for disruptive change from their technology choices. Aided by the framework, managers will be able to define the role their organisation can play in a public blockchain-based traceability solution. Furthermore, social entrepreneurs might find our framework helpful in developing traceability solutions to resolve social, cultural, or environmental issues without relying on intermediaries.
Much of the current research focuses on implementation with business benefits. However, the intermediary-free peer-to-peer design allows for disruptive innovations. We call on researchers to investigate traceability solutions based on our framework and thereby focussing on the value of disintermediation and decentralisation. Taking our findings into account, public blockchain traceability could be highly beneficial to organisations like social entrepreneurs, decentralised autonomous organisation, or bottom of the pyramid businesses and markets. Future research should identify the benefit of the proposed blockchain cases in contrast to more traditional designs like shared databases. Our Framework will be helpful to demonstrate this blockchain-driven differentiation.
Figures
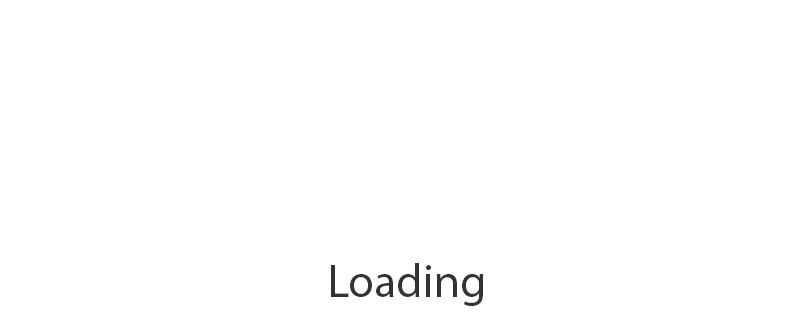
Figure 2
Blockchain privacy models based on Nakamoto (2008)
Traceability constructs
Construct | Definition | References |
---|---|---|
Unique identification | All objects like materials, components and assemblies uniquely are identifiable | Global Standards One (2017), Jansen-Vullers et al. (2003), Moe (1998), Opara and Mazaud (2001) |
Capture and recording | To be captured data and their structure are defined throughout the supply chain | Global Standards One (2017), Jansen-Vullers et al. (2003) |
Linking process and data | Transformational and custody information are linked to objects | Global Standards One (2017), Jansen-Vullers et al. (2003), Moe (1998), Opara and Mazaud (2001), Storøy et al. (2008) |
Communication and reporting | Data requirements regarding communication and reporting needs are defined | Bollen (2005), Biagioni et al. (2011), Global Standards One (2017), Jansen-Vullers et al. (2003), Skilton and Robinson (2009) |
Blockchain constructs
Construct | Definition | References |
---|---|---|
Disintermediation | Data integrity is through the network-wide consensus mechanisms, not a trusted 3rd party. Transactions are public and identities are protected | Nakamoto (2008); Narayanan et al. (2016) |
Transparency | Transactional transparency requires anonymity of identities to protect privacy Transactions transfer electronic assets, not real-world objects | Wang et al. (2020); Zheng et al. (2017); Scully and Hobig, (2019) |
Decentralisation | Decentralised data infrastructure creates robustness, security and availability. Participants free to join or withdraw from the network | Bogart and Rice (2015); Schollmeier (2002); Sompolinsky and Zohar (2013); Zheng et al. (2017) |
Immutability | Once validated, data and transactions are secured through the transaction chain | Nakamoto (2008); Pournader et al. (2020); Wang et al. (2020) |
Traceability perspective on the blockchain
Traceability construct | Blockchain |
---|---|
Unique identification |
|
Capture and recording |
|
Linking process and data |
|
Communication and reporting |
|
Blockchain perspective on the traceability
Blockchain construct | Supply chain traceability |
---|---|
Disintermediation |
|
Transparency |
|
Decentralisation |
|
Immutability |
|
References
Abeyratne, S.A. and Monfared, R.P. (2016), “Blockchain ready manufacturing supply chain using distributed ledger”, International Journal of Research in Engineering and Technology, Vol. 5 No. 9, pp. 1-10.
Abiteboul, S., Hull, R. and Vianu, V. (1996), Foundations of Databases, Reprinted with Corr, Addison-Wesley, Reading, Mass.
Aung, M.M. and Chang, Y.S. (2014), “Traceability in a food supply chain: safety and quality perspectives”, Food Control, Vol. 39, pp. 172-184.
Babich, V. and Hilary, G. (2020), “OM forum—distributed ledgers and operations: what operations management researchers should know about blockchain technology”, Manufacturing and Service Operations Management, Vol. 22 No. 2, pp. 223-240.
Bai, C. and Sarkis, J. (2020), “A supply chain transparency and sustainability technology appraisal model for blockchain technology”, International Journal of Production Research, Vol. 58 No. 7, pp. 2142-2162.
Bailey, M., Bush, S.R., Miller, A. and Kochen, M. (2016), “The role of traceability in transforming seafood governance in the global South”, Current Opinion in Environmental Sustainability, Vol. 18, pp. 25-32.
Bechini, A., Cimino, M.G.C.A., Marcelloni, F. and Tomasi, A. (2008), “Patterns and technologies for enabling supply chain traceability through collaborative e-business”, Information and Software Technology, Vol. 50 No. 4, pp. 342-359.
Behnke, K. and Janssen, M. (2019), “Boundary conditions for traceability in food supply chains using blockchain technology”, International Journal of Information Management, Vol. 52, 101969.
Biagioni, J., Gerlich, T., Merrifield, T. and Eriksson, J. (2011), “EasyTracker”, in Liu, J., Levis, P. and Römer, K. (Eds), Proceedings of the 9th ACM Conference on Embedded Networked Sensor Systems - SenSys '11, 01.11.2011 - 04.11.2011, Seattle, Washington, ACM Press, New York, NY, p. 68.
Blossey, G., Eisenhardt, J. and Hahn, G. (2019), “Blockchain technology in supply chain management: an application perspective”, Proceedings of the 52nd Hawaii International Conference on System Sciences (2019), Hawaii International Conference on System Sciences, pp. 6885-6893.
Bogart, S. and Rice, K. (2015), “The blockchain report: welcome to the internet of value”, Needham Insights, October 21, available at: https://docs.google.com/viewer?a=v&pid=sites&srcid=ZGVmYXVsdGRvbWFpbnxiaXRjb2luYW5kYmxvY2tjaGFpbnRvcmd8Z3g6NzZkOGY3ZDRkZWIxOWI2YQ (accessed 3 August 2021).
Bollen, A.F. (2005), “Traceability in fresh produce supply chains”, Acta Horticulturae, Vol. 687, pp. 279-288.
Bosona, T. and Gebresenbet, G. (2013), “Food traceability as an integral part of logistics management in food and agricultural supply chain”, Food Control, Vol. 33 No. 1, pp. 32-48.
Bosona, T., Gebresenbet, G. and Olsson, S.O. (2018), “Traceability system for improved utilization of solid biofuel from agricultural prunings”, Sustainability, Vol. 10 No. 2, p. 258.
Casino, F., Dasaklis, T.K. and Patsakis, C. (2019), “A systematic literature review of blockchain-based applications: current status, classification and open issues”, Telematics and Informatics, Vol. 36, pp. 55-81.
Chang, Y., Iakovou, E. and Shi, W. (2020), “Blockchain in global supply chains and cross border trade: a critical synthesis of the state-of-the-art, challenges and opportunities”, International Journal of Production Research, Vol. 58 No. 7, pp. 2082-2099.
Chaudhuri, A., Dukovska-Popovska, I., Subramanian, N., Chan, H.K. and Bai, R. (2018), “Decision-making in cold chain logistics using data analytics: a literature review”, The International Journal of Logistics Management, Vol. 29 No. 3, pp. 839-861.
Choi, T.M., Feng, L. and Li, R. (2020), “Information disclosure structure in supply chains with rental service platforms in the blockchain technology era”, International Journal of Production Economics, Vol. 221, 107473.
Cole, R., Stevenson, M. and Aitken, J. (2019), “Blockchain technology: implications for operations and supply chain management”, Supply Chain Management: An International Journal, Vol. 24 No. 4, pp. 469-483.
Diffie, W. and Hellman, M. (1976), “New directions in cryptography”, IEEE Transactions on Information Theory, Vol. 22 No. 6, pp. 644-654.
Digital Transformation Agency (2019), “Blockchain overview: Australian Government guide”, available at: https://www.dta.gov.au/help-and-advice/technology/blockchain/blockchain-overview-australian-government-guide.
DuPont, Q. (2018), “Social and technical opportunities and risks of cryptocurrencies and blockchains”, Presented to Committee on Science, Technology, and Law, National Academies of Sciences, Engineering, and Medicine, available at: https://ssrn.com/abstract=3267514 (accessed 3 August 2021).
Fridgen, G., Prinz, W., Rose, T. and Urbach, N. (2017), “Blockchain lab‐design, implementation and evaluation of innovative business and process models”, Blockchain Engineering, Vol. 110, p. 36.
Fritz, M. and Schiefer, G. (2009), “Tracking, tracing, and business process interests in food commodities: a multi-level decision complexity”, International Journal of Production Economics, Vol. 117 No. 2, pp. 317-329.
Garcia-Torres, S., Albareda, L., Rey-Garcia, M. and Seuring, S. (2019), “Traceability for sustainability‐literature review and conceptual framework”, Supply Chain Management: An International Journal, Vol. 24 No. 1, pp. 85-106.
Garrard, R. and Fielke, S. (2020), “Blockchain for trustworthy provenances: a case study in the Australian aquaculture industry”, Technology in Society, Vol. 62, 101298.
Global Standards One (2017), “Global standards one: GS1 global traceability standard. Framework for the design of interoperable traceability systems for supply chains”, Release 2.0, Ratified, Aug 2017, available at: https://www.gs1.org/sites/default/files/docs/traceability/GS1 (accessed 3 August 2021).
Haber, S. and Stornetta, W.S. (1991), “How to time-stamp a digital document”, Journal of Cryptology, Vol. 3 No. 2, pp. 99-111.
Hald, K.S. and Kinra, A. (2019), “How the blockchain enables and constrains supply chain performance”, International Journal of Physical Distribution and Logistics Management, Vol. 49 No. 4, pp. 376-397.
Hastig, G.M. and Sodhi, M.S. (2020), “Blockchain for supply chain traceability: business requirements and critical success factors”, Production and Operations Management, Vol. 29 No. 4, pp. 935-954.
Huang, G.Q., Lau, J.S.K. and Mak, K.L. (2003), “The impacts of sharing production information on supply chain dynamics: a review of the literature”, International Journal of Production Research, Vol. 41 No. 7, pp. 1483-1517.
Jansen-Vullers, M.H., van Dorp, C.A. and Beulens, A.J.M. (2003), “Managing traceability information in manufacture”, International Journal of Information Management, Vol. 23 No. 5, pp. 395-413.
Karippacheril, T.G., Rios, L.D. and Srivastava, L. (2017), “Global markets, global challenges: improving food safety and traceability while empowering smallholders through ICT”, in ICT in Agriculture (Updated Edition): Connecting Smallholders to Knowledge, Networks, and Institutions, The World Bank, Vol. 41, pp. 283-308.
Kim, H.M., Fox, M.S. and Gruninger, M. (1995), “An ontology of quality for enterprise modelling”, in Kavanaugh, M.E. (Ed.), Proceedings of the Fourth Workshop on Enabling Technologies: Infrastructure for Collaborative Enterprises: April 20-22, 1995, Berkeley Springs, West Virginia, 20-22 April 1995, Berkeley Springs, WV, USA, IEEE Computer Soc. Press, LosAlamitos, California, CA, pp. 105-116.
Koh, L., Dolgui, A. and Sarkis, J. (2020), “Blockchain in transport and logistics – paradigms and transitions”, International Journal of Production Research, Vol. 58 No. 7, pp. 2054-2062.
Kolk, A. (2008), “Sustainability, accountability and corporate governance: exploring multinationals' reporting practices”, Business Strategy and the Environment, Vol. 17 No. 1, pp. 1-15.
Korpela, K., Hallikas, J. and Dahlberg, T. (2017), “Digital supply chain transformation toward blockchain integration”, Proceedings of the 50th Hawaii International Conference on System Sciences (2017), Hawaii International Conference on System Sciences.
Kshetri, N. (2018), “Blockchain's roles in meeting key supply chain management objectives”, International Journal of Information Management, Vol. 39, pp. 80-89.
Lao, L., Li, Z., Hou, S., Xiao, B., Guo, S. and Yang, Y. (2020), “A survey of IoT applications in blockchain systems”, ACM Computing Surveys, Vol. 53 No. 1, pp. 1-32.
Lu, Q., Xu, X., Liu, Y. and Zhang, W. (2018), “Design pattern as a service for blockchain applications”, in Tong, H. (Ed.), 18th IEEE International Conference on Data Mining Workshops: Proceedings 17–20 November 2018, Singapore, 11/17/2018 - 11/20/2018, Singapore, Singapore, IEEE Computer Society, Conference Publishing Services, Los Alamitos, California, CA, pp. 128-135.
Manupati, V.K., Schoenherr, T., Ramkumar, M., Wagner, S.M., Pabba, S.K. and Inder Raj Singh, R. (2019), “A blockchain-based approach for a multi-echelon sustainable supply chain”, International Journal of Production Research, Vol. 58 No. 7, pp. 2222-2241.
Merkle, R. (1979), Secrecy, Authentication, and Public Key Systems, Ph. D. Thesis, Stanford University, Stanford, CA, available at: https://www.merkle.com/papers/Thesis1979.pdf (accessed 3 August 2021).
Min, H. (2019), “Blockchain technology for enhancing supply chain resilience”, Business Horizons, Vol. 62 No. 1, pp. 35-45.
Moe, T. (1998), “Perspectives on traceability in food manufacture”, Trends in Food Science and Technology, Vol. 9 No. 5, pp. 211-214.
Nakamoto, S. (2008), “Bitcoin: a peer-to-peer electronic cash system”, available at: https://bitcoin.org/en/bitcoin-paper (accessed 3 August 2021).
Narayanan, A. (2015), “Private blockchain' is just a confusing name for a shared database, Retrieved May, Vol. 3, p. 2018.
Narayanan, A., Bonneau, J., Felten, E., Miller, A. and Goldfeder, S. (2016), Bitcoin and Cryptocurrency Technologies: A Comprehensive Introduction, Princeton University Press, Princeton, NJ.
Opara, L.U. and Mazaud, F. (2001), “Food traceability from field to plate”, Outlook on Agriculture, Vol. 30 No. 4, pp. 239-247.
Pournader, M., Shi, Y., Seuring, S. and Koh, S.L. (2020), “Blockchain applications in supply chains, transport and logistics: a systematic review of the literature”, International Journal of Production Research, Vol. 58 No. 7, pp. 2063-2081.
Prajogo, D. and Olhager, J. (2012), “Supply chain integration and performance: the effects of long-term relationships, information technology and sharing, and logistics integration”, International Journal of Production Economics, Vol. 135 No. 1, pp. 514-522.
Qiao, R., Zhu, S., Wang, Q. and Qin, J. (2018), “Optimization of dynamic data traceability mechanism in internet of things based on consortium blockchain”, International Journal of Distributed Sensor Networks, Vol. 14 No. 12, 155014771881907.
Queiroz, M.M., Telles, R. and Bonilla, S.H. (2019), “Blockchain and supply chain management integration: a systematic review of the literature”, Supply Chain Management: An International Journal, Vol. 12 No. 1, p. 671.
Rao, B.S. and Durrant-Whyte, H.F. (1991), “Fully decentralised algorithm for multisensor Kalman filtering”, IEE Proceedings D Control Theory and Applications, Vol. 138 No. 5, p. 413.
Rao, B.S.Y., Durrant-Whyte, H.F. and Sheen, J.A. (1993), “A fully decentralized multi-sensor system for tracking and surveillance”, The International Journal of Robotics Research, Vol. 12 No. 1, pp. 20-44.
Rodrigo, M.N.N., Perera, S., Senaratne, S. and Jin, X. (2018), “Blockchain for construction supply chains: a literature synthesis”, Proceedings of ICEC-PAQS Conference.
Saberi, S., Kouhizadeh, M., Sarkis, J. and Shen, L. (2019), “Blockchain technology and its relationships to sustainable supply chain management”, International Journal of Production Research, Vol. 57 No. 7, pp. 2117-2135.
Schollmeier, R. (2002), “A definition of peer-to-peer networking for the classification of peer-to-peer architectures and applications”, in Graham, R.L. and Shahmehri, N. (Eds), First International Conference on Peer-To-Peer Computing: Proceedings, 27-29 August 2001, Linköping, Sweden, 27–29 Aug. 2001, IEEE Computer Society; IEEE, Los Alamitos, Calififornia, CA, Linkoping, pp. 101-102.
Scully, P. and Hobig, M. (2019), “Exploring the impact of blockchain on digitized supply chain flows: a literature review”, 2019 Sixth International Conference on Software Defined Systems (SDS): 10–13 June, 2019, Rome, Italy, 6/10/2019 - 6/13/2019, IEEE, [Piscataway, New Jersey], pp. 278-283.
Skilton, P.F. and Robinson, J.L. (2009), “Traceability and normal accident theory: how does supply network complexity influence the traceability of adverse events?”, Journal of Supply Chain Management, Vol. 45 No. 3, pp. 40-53.
Sompolinsky, Y. and Zohar, A. (2013), “Accelerating bitcoin's transaction processing”, Fast Money Grows on Trees, Not Chains, IACR Cryptol., ePrint Arch, pp. 1-28.
Storøy, J., Senneset, G., Forås, E., Olsen, P., Karlsen, K.M. and Frederiksen, M.T. (2008), “Improving traceability in seafood production”, in Børresen, T. (Ed.), Improving Seafood Products for the Consumer, British Welding Research Association, Cambridge, pp. 516-538.
Szabo, N. (1994), “Smart contracts”, available at: http://www.fon.hum.uva.nl/rob/Courses/InformationInSpeech/CDROM/Literature/LOTwinterschool2006/szabo.best.vwh.net/smart.contracts.html (accessed 3 December 2018).
Tapscott, D. and Tapscott, A. (2016), Blockchain Revolution: How the Technology behind Bitcoin Is Changing Money, Business, and the World, International ed., Portfolio/Penguin, New York, NY.
Tatar, J. (2019), “How blockchain technology is revolutionary”, available at: https://www.thebalance.com/how-revolutionary-is-blockchain-technology-4045555.
van Dorp, K.‐J. (2002), “Tracking and tracing: a structure for development and contemporary practices”, Logistics Information Management, Vol. 15 No. 1, pp. 24-33.
van Hoek, R. (2019), “Developing a framework for considering blockchain pilots in the supply chain – lessons from early industry adopters”, Supply Chain Management: An International Journal, Vol. 25 No. 1, pp. 115-121.
Voshmgir, S. (2017), “Disrupting governance with blockchains and smart contracts”, Strategic Change, Vol. 26 No. 5, pp. 499-509.
Wang, Y., Han, J.H. and Beynon-Davies, P. (2019a), “Understanding blockchain technology for future supply chains: a systematic literature review and research agenda”, Supply Chain Management: An International Journal, Vol. 24 No. 1, pp. 62-84.
Wang, Y., Singgih, M., Wang, J. and Rit, M. (2019b), “Making sense of blockchain technology: how will it transform supply chains?”, International Journal of Production Economics, Vol. 211, pp. 221-236.
Wang, M., Asian, S., Wood, L.C. and Wang, B. (2020), “Logistics innovation capability and its impacts on the supply chain risks in the Industry 4.0 era”, Modern Supply Chain Research and Applications, Vol. 2 No. 2, pp. 83-98.
Yaga, D., Mell, P., Roby, N. and Scarfone, K. (2018), Nistir 8202 Blockchain Technology Overview, National Institute of Standards and Technology, available at: https://nvlpubs. nist. gov/nistpubs/ir/2018/NIST (accessed 3 August 2021).
Zheng, Z., Xie, S., Dai, H., Chen, X. and Wang, H. (2017), “An overview of blockchain technology: architecture, consensus, and future trends”, in Karypis, G. and Zhang, J. (Eds), 2017 IEEE International Congress on Big Data - BigData Congress 2017: 25-30 June 2017, Honolulu, Hawaii, USA Proceedings, 6/25/2017 - 6/30/2017, Honolulu, HI, USA, IEEE, Piscataway, New Jerssey, NJ, pp. 557-564.