Abstract
Purpose
The purpose of this study is to evaluate the vulnerability of the important rangeland shrub, Atriplex leucoclada (Boiss) to both climate change and livestock grazing, within the Syrian rangelands as a representative landscape type of West Asia.
Design/methodology/approach
Ecologically based quantitative niche models were developed for both shrub species using maximum entropy and 13 spatially explicit GIS-based layers to predict current and future species distribution scenarios. Climatic variables varied over time in line with the predictions created from the HADCM3 global circulation model.
Findings
Results indicate that with grazing and climate change, the distribution of A. leucoclada will be reduced by 54 per cent in 2050, with the mean annual and minimum temperatures of the coldest month having the highest contribution in the model (28.7 and 21.2 per cent, respectively). The contribution of the grazing pressure, expressed by the overgrazing index, was estimated at 8.2 per cent.
Originality/value
These results suggest that the interaction of climate and increased grazing has the potential to favor the establishment of unpalatable species, while reducing the distribution of preferred plant species on western Asia rangelands.
Keywords
Citation
Louhaichi, M., Ouled Belgacem, A., Petersen, S.L. and Hassan, S. (2019), "Effects of climate change and grazing pressure on shrub communities of West Asian rangelands", International Journal of Climate Change Strategies and Management, Vol. 11 No. 5, pp. 660-671. https://doi.org/10.1108/IJCCSM-02-2018-0017
Publisher
:Emerald Publishing Limited
Copyright © 2019, Mounir Louhaichi, Azaiez Ouled Belgacem, Steven L. Petersen and Sawsan Hassan.
License
Published by Emerald Publishing Limited. This article is published under the Creative Commons Attribution (CC BY 4.0) licence. Anyone may reproduce, distribute, translate and create derivative works of this article (for both commercial & non-commercial purposes), subject to full attribution to the original publication and authors. The full terms of this licence may be seen at http://creativecommons.org/licences/by/4.0/legalcode
1. Introduction
Climate change has been considered a primary global environmental threat that will limit the distribution and abundance of plant populations worldwide (Pearson and Dawson, 2003). Research suggests that by 2100, impacts related to climate change may be the primary causal factor of the decline in global biodiversity and ecosystem services (Metzger et al., 2006; Phillips et al., 2006). This loss in biodiversity may be attributed to the inability of native plants to survive changes in climate patterns, such as poor adaptability of some species to seasonal fluctuations in temperature and precipitation (Ouled Belgacem and Louhaichi, 2013).
Climate change scenarios developed by the Intergovernmental Panel on Climate Change (IPCC) identify a steady increase in global mean surface temperature of 2°C-6°C above pre-industrial levels by 2100 (IPCC, 2007). In response to this increase, climate change scenarios predict greater fluctuations in global mean soil surface temperatures and temporal precipitation patterns that may induce more severe flooding and prolonged drought patterns, impacting ecosystem services at global scales (IPCC, 2007). In recent years, the ecological consequences of ongoing climate change patterns have been debated (Ouled Belgacem and Louhaichi, 2013). However, a body of recently published literature warn of the potentially negative effects of climate change on plant community dynamics and ecosystem sustainability, particularly when coupled with impacts resulting from human-related activities (i.e. grazing) (Freier et al., 2011; Ouled Belgacem and Louhaichi, 2013).
Rangelands are highly susceptible to the impacts of climate change in response to limited water availability and higher air and soil temperatures (Ouled Belgacem and Louhaichi, 2013). Impacts on rangeland vegetation include reduced growth rates, lower photosynthetic rates, impaired mineral absorption, low tissue regeneration and increased concentrations of secondary metabolites such as ginsenosides and polyphenols (Jochum et al., 2007). The reduced vegetation cover and lower plant biodiversity increase the susceptibility of rangelands to projected fluctuations in climate change (Ouled Belgacem and Louhaichi, 2013; Hudson et al., 2014). This is often accentuated by shallow soils with low nutrient content that limits plant growth and cover and increases erosion potential (Hudson et al., 2014).
West Asia rangelands represent a large and ecologically important land-use type that are characterized by arid or semi-arid climates with low and unpredictable precipitation, as well as variable air and soil temperature patterns (Louhaichi et al., 2016a). These lands provide a diversity of ecological services (i.e. nutrient cycling, pollution filtering, soil stabilization, the preservation of unique plants and animals) that are socially and economically important, providing an important forage resource for livestock production and sustaining local pastoral communities and culture (Louhaichi et al., 2016b). Complex political, socio-economical and environmental interactions have led to the progressive degradation of West Asia rangelands over the past century (Hudson et al., 2014). This degradation has been exacerbated by poor rangeland planning, insufficient implementation of appropriate land management practices and the unsustainable use of limited resources (i.e. water, forage and soil; Hudson et al., 2014). Additionally, these impacts have been amplified by harsh environmental conditions such as frequent and prolonged drought (Louhaichi and Tastad, 2010).
For evaluating and predicting rangeland plant establishment and distribution patterns related to climate change related variables, ecological niche models (ENMs) are a valuable tool (Guisan and Zimmermann, 2000). ENMs examine the association between species occurrence and any number of associated biotic and abiotic environmental variables within a specified area and are then applied broadly to yield estimates for comparison with suitable environmental conditions (Peterson et al., 2007, 2011; Shcheglovitova and Anderson, 2013). ENMs help land managers identify environmental conditions that facilitate the establishment and survival of rangeland plant species in response to climate change (Guisan and Zimmermann, 2000). Such changes include fluctuations in temperature, limitations in water availability and changes in climate-soil microsites that all lead to greater susceptibility, lower establishment and decreased plant survival rates (Schneider et al., 2007).
A suite of tools has been developed to evaluate and prioritize management actions in response to different climate change scenarios, such as current and historic distribution patterns (Millar et al., 2007). These output data are then used to guide the development of new policy that would help mitigate the actual or anticipated impacts on biodiversity for a particular area (Fitzpatrick et al., 2008). For example, climate envelope modeling rapidly assesses the potential impact of climate change on species spatial distribution (Hijmans and Graham, 2006). Climate envelope models use the geographic distribution of a represented species for predicting the probable occurrence of a particular climatic niche (Pearson and Dawson, 2003). These tools can help managers assess risk and identify land management practices or technologies that improve plant establishment potential (Hijmans and Graham, 2006; Pearson and Dawson, 2003).
The Syrian rangelands play an important role for sustaining the local human populations and maintaining ecological sustainability (Bryceson, 2003). The shift in plant community composition in such rangelands may have a negative impact on livestock grazing, and, as such, the purpose of this study is to assess the effect of climate change and grazing pressure on the geographical distribution of Atriplex leucoclada in the Syrian rangelands. This study also seeks to characterize the potential effect this distribution may have on current and future rangeland structure and therefore associated livestock grazing.
2. Materials and methods
2.1 Study area
The Syrian rangelands, considered as a representative landscape type of western Asia, cover over 10 million ha, or 55 per cent of the country’s land area, including large regions of central and eastern Syria (Louhaichi and Tastad, 2010). Soils are classified as Aridisols with distinct calcic or gypsic horizons near the soil surface (Louhaichi and Tastad, 2010). These soils have weak structure and a relatively light texture, which predisposes them to potentially higher rates of erosion (ACSAD, 2004). The Syrian rangelands are characterized by low rainfall (less than 200 mm per year), which decreases from west to east (Al-Bakri et al., 2001). The local communities that use rangelands for raising livestock herd approximately 12 million animals (ACSAD, 2004). Livestock raised in these lands are predominantly sheep (10 million), followed by goats (about 1.6 million) and camels (about 27,000; ACSAD, 2004). Stocking rates have typically exceeded carrying capacity which has led to drastic deterioration of native plant communities and the inability to sustain successful animal production systems (Louhaichi et al., 2012). For this study, we focused on the central region of Syrian rangelands (2.8 million ha or approximately 30 per cent of the total area).
2.2 Target range species
A. leucoclada is an important native rangeland plant species that range from West Asia to Egypt (Le Houérou, 1996). A. leucoclada grows in dry and desert areas of West Asia rangelands (Post and Dinsmore, 1933). It is drought-resistant and halophytic, tolerating soil salinity levels as high as 30 dS/m (Al-Oudat and Qadir, 2011). The plant exhibits high forage value and is grazed by all livestock classes (Murad, 1996). A. leucoclada has been shown to readily establish from seed (Le Houérou, 1996), thus enhancing restoration efforts (Murad, 1996).
2.3 Environmental and socioeconomic data
Environmental and grazing variables were used to develop niche models which were the same as those used by Ouled Belgacem and Louhaichi (2013) in a previous study. They included eight climatic layers, three soil property layers, one altitude layer and one grazing pressure layer. The eight climate variables included mean annual temperature, minimum temperature of the coldest month, maximum temperature of the warmest month, seasonal temperature variation, mean annual precipitation, precipitation of the wettest month, precipitation of the driest month and seasonal precipitation variation. These variables were developed as GIS-based data layers provided by the world climate database (www.worldclim.org; Hijmans et al., 2005), with a 2.5 arcmin pixel size. Climatic variables also included altitude and the distribution area for each shrub species.
In addition to current climate estimates, the change in climate patterns over time for each variable was modeled for the year 2050. These were based on predictions created from the global circulation model HADCM3 that uses the frame of an A2 CO2 emissions scenario (IPCC, 2000). A2 “storyline” scenarios describe heterogeneous environments with an underlying theme of self-reliance and preservation of local human identities. Human fertility patterns across these regions slowly converge, resulting in continuously increasing populations that contribute to increased CO2 emissions. Economic development is regionally oriented, and per capita economic growth and technological change are more fragmented and slower than other storylines. The A2 emissions scenario was chosen as it was one of the “marker” scenarios developed through the IPCC. The A2 scenario is at the higher end of the Special Report on Emissions Scenarios preferred because, from an impact and adaptation point of view, if one can adapt to a larger climate change, then adaptation to smaller climate changes of the lower end scenarios are expected. These data (grid format) were downloaded from the gisweb database (www.gisweb.ciat.cgiar.org), also with 2.5 arcmin resolution.
The three soil variables included soil texture (percentage of sand, silt and clay), soil depth and soil salinity. These variables are considered critical for accurately mapping the spatial distribution of individual plant species within arid areas (Florêt and Pontanier, 1982). These layers were extracted from the Badia Development Project (ACSAD, 2004). A socioeconomic parameter was used to assess the influence of anthropogenic pressures that contribute to plant cover and species composition changes in addition to environmentally associated climatic factors.
Livestock grazing is the primary anthropogenic factor that influences species vulnerability, ecological degradation, extirpation of species, and low productivity (O’Brien et al., 2004). The socioeconomic layer developed for this study is represented by a synthetic variable, the coefficient of overgrazing (CO), which reflects the current condition of the species in relation to grazing intensity (Ouled Belgacem and Louhaichi, 2013). To distinguish the influence of climate change from grazing, grazing pressure remained constant over time. Grazing intensity data sets were generated from both current grazing capacity (GC) and stocking density (SD) (expressed as the spatial distribution of herds managed within the study area) (ACSAD, 2004). It was determined based on the following formula (Le Houérou, 1980):
2.4 Climate envelope and species spatial distribution modeling
Climate envelope models are used to predict future shifts in a climatic niche which, in turn, estimate climate projections provided by global circulation models (Hijmans and Graham, 2006). To meet our objective, we applied the ecological niche concept (Elith et al., 2006, 2011; Phillips et al., 2006) to establish a relationship between species occurrence and environmental factors. We used maximum entropy (MaxEnt) to model species distribution, as it can accurately model species spatial distribution for both present (Elith et al., 2006, 2011; Ouled Belgacem and Louhaichi, 2013) and future environmental conditions (Hijmans and Graham, 2006). MaxEnt allows for multiple iterations of model development that are then averaged (Phillips et al., 2006). Contrary to other modeling techniques, which focus on model development using presence/absence or abundance data, MaxEnt uses presence-only occurrence data (Phillips et al., 2006), with a strong focus on the role of regularization in parameter estimation. Regularization has the most impact when sample sizes are small, subsequently MaxEnt regularizes in relation to sample size (Phillips et al., 2006). In this case, only presence maps of both target species were produced using 2004 ACSAD (Figure 1).
Withholding a select portion of the data enables testing of model performance while taking advantage of all available data without requiring an independent data set. Executing multiple runs also provides a measure of the amount of variability in the model. In our study, we chose the auto features (linear, quadratic product, threshold and hinge features) function and selected the output format as Logistic and output file type as “.asc”. Parameters used in selection setting included,
a random test percentage of “null”;
no random seed; and
a maximum number of iterations set at 500.
Model validation was conducted by comparing maps predicting current species vulnerability with actual distribution.
3. Results
Under current favorable environmental conditions and when protected from heavy grazing, A. leucoclada establishes and occupies intact West Asia rangelands over time (Figure 2). However, when change in climate is coupled with heavy livestock grazing, the distribution of A. leucoclada is expected to be reduced to more than 54 per cent by 2050. Vulnerability was greatest in the 2050 model, which was characterized by a high number of small, restricted and scattered patches of A. leucoclada dominated sites. In this analysis, we used the per cent contribution, an estimate of the relative contribution of each variable to the MaxEnt model. Per cent contribution considers each environmental and socioeconomic variable for characterizing trends (Table I). Among the environmental variables, those that were found to most restrict the geographical distribution of A. leucoclada included:
mean annual temperature (28.7 per cent contribution);
minimum temperature of the coldest month (21.2 per cent);
mean annual precipitation (14.1 per cent); and
maximum temperature of the warmest month (11.7 per cent).
Grazing pressure contributed 8.2 per cent to the model, suggesting a negative effect of grazing and climate change on species and plant community resilience (recovery potential of a plant community; Briske et al., 2005). The other variables associated with landform, i.e. geomorphology and soil conditions, present at the site contributed little in explaining species distribution trends and patterns.
4. Discussion
The impact of climate change on rangeland ecosystems has been predicted to impair vegetation composition, plant establishment and plant community resilience (McKeon et al., 2009; Thornton et al., 2009). Results of this study indicate that A. leucoclada, an ecologically important rangeland species, exhibits sensitivity to increased temperature regimes and decreased precipitation levels. For example, plant emergence and establishment of A. leucoclada are limited by low soil moisture levels, a trait that is expected with current and future climate change scenarios. An increase in air temperature may limit plant emergence and survival by reducing available soil moisture and increasing plant evapotranspiration, particularly during the first and second year following seedling establishment. These results are supported by Assaeed et al. (2012), who reported a significant decrease in seedling survival during the summer months, when annual temperatures were highest.
Vulnerability of A. leucoclada to climate change is explained by not only its sensitivity to increased air and soil temperatures or by the reduction in total precipitation but also the influence of high grazing pressure and the subsequent reduction in the adaptive capacity of A. leucoclada. It has been shown that drought combined with high grazing pressure are convergent forces that are particularly detrimental to palatable perennial species, including A. leucoclada (Quiroga et al., 2010). Overgrazing of desirable rangeland species impacts plant community composition and cover, potentially influencing ecological processes and their recovery following disturbance (i.e. reduced ecological resilience; Briske et al., 2005, 2008). Furthermore, appropriate selection of grazing strategies is fundamental for predicting potential effects of grazing on plant communities, particularly with increasing temperature and decreasing precipitation trends (Fuhlendorf and Engle, 2001). Continuous grazing with no, or limited, management would likely have significant negative long-term effects on the occurrence and distribution of A. leucoclada. In contrast, carefully applied management that controls livestock stocking rates, grazing duration and the period (season) of grazing would be important considerations for reducing the impacts of climate change on grazing carrying capacity and species distribution (Holochek et al., 2004).
In contrast, previous modeling of non-palatable Syrian rangeland species such as Haloxylon salicornicum (Moq.) Bunge, a xerophilous species that is known for its adaptation to drought and higher temperatures, suggested an increase of its geographic distribution with climate change (Ouled Belgacem and Louhaichi, 2013). This drought-tolerant species also maintains tolerance to a wide range of soil properties including soil salinity, low nutrient content and eolian movement (Salama et al., 2005). Additionally, it has a low sensitivity to drought, salinity, poor nutrition, sand movement and high light intensity, because of the broad ecological niche expected for this species (Huang et al., 2003). As a consequence, the increase in H. salicornicum distribution could result in a shift in plant composition and dominance, potentially resulting in impaired ecological processes and the potential transition across ecological thresholds (Stringham et al., 2003; Petersen et al., 2009). Such increases, which result from the interaction of livestock grazing and climate change, could alter the distribution and biodiversity of rangeland plant communities as evidenced in this study for the year 2050 (Guisan and Theurillat, 2000; Guma et al., 2010). As such, the influence of increasing temperatures and lower precipitation could create conditions that favor invading species such as H. salicornicum at the expense of the high range value species such as A. leucoclada, particularly when considering rangelands with low ecological resilience (Briske et al., 2008).
In rangelands, there has been a shift from the nomadic or pastoral way of life to a more sedentary agro-pastoral production system, with extensive livestock production representing the main component of the agricultural economy of these agro-pastorals (FAO, 2010). This increase in livestock demand has intensified grazing pressure on natural resources, in particular natural vegetation cover and biomass (Lemaire et al., 2014). This shift from nomadic to sedentary production systems has led to the expansion of cultivation into the best rangeland sites having deeper soil and higher organic matter (Nefzaoui et al., 2014). Consequently, grazing lands cover only a small portion of the livestock feeding need (Nordblom, 1992). Thus, there is a negative impact on agriculture when rangelands are degraded. The more degraded the rangelands, the more the pressure will be on croplands (Reitsma et al., 2015). As such, increased pressure on grazing land substantiates the model’s findings that an increase in H. salicornicum is a more likely scenario, owing to the increased human population resulting in increased demand for livestock products and agricultural land (FAO, 2010).
Model validation was achieved using a specified number of iterations or training points. This, as is the case in other models, may give new predicted areas of occurrence for the studied species, and these are clearly among the most relevant outputs of ecological modeling (Hernandez et al., 2006). However, new areas of predicted occurrence are rarely verified in the field (Rebelo and Jones, 2010) which is of concern, especially for areas outside the known geographical range of the species (Elith et al., 2006). Such a lack of validation is highly relevant for rare or closely rare species like A. leucoclada, which are usually a conservation priority, but with a wider knowledge gap compared to more common and abundant species.
Considering these impacts and limitations, ecological niche modeling can be an excellent tool for obtaining approximate impacts of climate change and for assessing the potential for species impacts from plant invasions, as well as grazing intensities (Pearson and Dawson, 2003). These models can improve conservation and restoration efforts by aiding managers in locating sites with the highest potential for impacts (i.e. risk assessment) based on different climate change scenarios, interacting with utilization intensities of such important rangeland species. Consequently, a mitigation strategy to increase the resilience of the most vulnerable species, based on proper grazing, the selection of more drought tolerant taxa and the establishment of improved water harvesting techniques, needs to be developed and applied within West Asia rangelands.
5. Conclusion and management implications
The results of this study demonstrate that restoration and rehabilitation of degraded rangelands may be impaired by the combined effects of climate change and human-related activities (i.e. grazing pressure). These elements may result in altered ecosystem structure and function that can potentially impact the establishment of native desirable species, while facilitating invasive species. The risk from altered climate change scenarios on rangeland plant species depends on the sensitivity of the species to environmental change, change to host ecosystem structure and the vulnerability of already existing native species.
Research of arid land reclamation emphasizes poor establishment of native species and dominance of non-native/invasive species. This pattern continues to provide one of the greatest challenges to the management of arid rangeland landscapes, demonstrated by reduced ecological resilience and the potential transition of landscapes across ecological thresholds that are dominated by undesirable plant communities and slow ecological processes. This research uses geo-spatial technology and ecological modeling to assess site condition related to plant adaptability and then projects plant community dynamics in relation to global climate change scenarios. The unique contribution provided in this paper is the application of models for characterizing the suitability of two arid shrub species which are well adapted to the current and future climatic patterns. This information can be used by managers to determine the risk associated with change in current vegetation distribution of desired native plant species because of over utilization and to predict how these changes will impact rangeland ecosystem structure over time.
Although results from these models need to be cautiously interpreted, in particular because of the assumptions that underlie both model type and data used, the impacts described from these models warrant a clearer focus on how monitoring across a species’ range can provide an early detection system for identifying potential climate change impacts. To validate and further refine these models, additional studies are needed that investigate the physiological thresholds, because of climate change scenarios, as well as disturbance intensities, for individual species of interest or value.
Figures
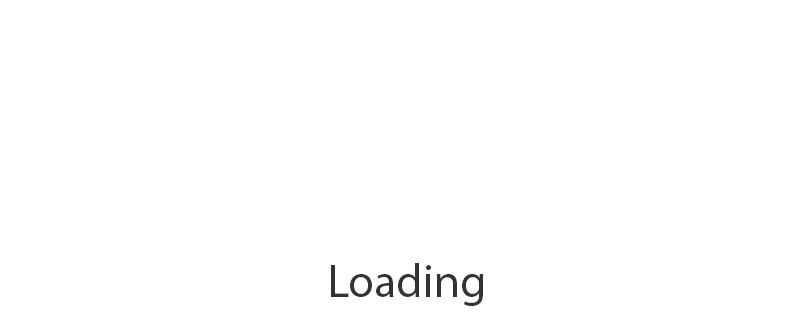
Figure 2.
Potential geographic distribution of Atriplex leucoclada in Syrian rangelands under present climate and the HADCM3 global circulation models for 2050. MaxEnt produces a continuous prediction with values ranging from 0 (blue) to 1 (red). The higher the predicted value the more suitable the environmental conditions for the species. In the current situation map, white pixels represent random training sites generated by the model for calibration purposes
Per cent contribution of environmental and socioeconomic variables in explaining the current and predicted geographic distribution of A. leucoclada located in the Syrian rangelands
Variables | Values |
---|---|
Environmental variables | |
Precipitation of wettest month | 14.1 |
Minimum temperature of coldest month | 21.2 |
Precipitation seasonality | 0 |
Temperature seasonality | 9.3 |
Annual precipitation | 2.8 |
Annual mean temperature | 28.7 |
Precipitation of driest month | 0 |
Maximum temperature of warmest month | 11.7 |
Altitude | 1.4 |
Soil depth | 0.5 |
Soil texture | 0 |
Soil salinity | 2.1 |
Socioeconomic variable | |
Coefficient of overgrazing | 8.2 |
References
Al-Bakri, J.T., Taylor, J.C. and Brewer, T.R. (2001), “Monitoring land use change in the Badia transition zone in Jordan using aerial photography and satellite imagery”, The Geographical Journal, Vol. 167 No. 3, pp. 248-262.
Al-Oudat, M. and Qadir, M. (2011), The Halophytic Flora of Syria, International Center for Agricultural Research in the Dry Areas, Aleppo, p. 186.
Arab Center for the Study of Arid Zones and Dry Lands (ACSAD) (2004), A Survey of the Natural Resources of the Syrian Steppe, ACSAD, Damascus.
Assaeed, A.M., El-Bana, M.I., Al-Doss, A.A. and Al-Mohaisen, I.A. (2012), “Forage yield and survival of native range species under supplementary irrigation in Central Saudi Arabia”, African Journal of Agricultural Research, Vol. 7 No. 27, pp. 3933-3938.
Briske, D.D., Fuhlendorf, S.D. and Smeins, F.E. (2005), “State-and-transition models, thresholds, and rangeland health: a synthesis of ecological concepts and perspectives”, Rangeland Ecology and Management, Vol. 58, pp. 1-10.
Briske, D.D., Bestelmeyer, B.T., Stringham, T.K. and Shaver, P.L. (2008), “Recommendations for development of resilience-based state-and-transition models”, Rangeland Ecology and Management, Vol. 61, pp. 359-367.
Bryceson, D.F. (2003), “Tenure, livelihoods and sustainable development – rangelands as sys-tems for multiple land use and livelihood support”, African Journal of Range and Forage, Science, Vol. 20 No. 2, pp. 202-209.
Elith, J., Steven, J., Phillips, S.J., Hastie, T., Dudik, M., Chee, Y.E. and Yates, C.J. (2011), “A statistical explanation of MaxEnt for ecologists”, Diversity and Distributions, Vol. 17 No. 1, pp. 43-57.
Elith, J., Graham, C.H., Anderson, R.P., Dudík, M., Ferrier, S., Guisan, A., Hijmans, R.J., Huettmann, F., Leathwick, J.R., Lehmann, A., Li, J., Lohmann, L.G., Loiselle, B.A., Manion, G., Moritz, C., Nakamura, M., Nakazawa, Y., Overton, J., McC, Peterson, A.T., Phillips, S.J., Richardson, K.S., Scachetti-Pereira, R., Schapire, R.E., Soberón, J., Williams, S., Wisz, M.S. and Zimmermann, N.E. (2006), “Novel methods improve prediction of species’ distributions from occurrence data”, Ecography, Vol. 29 No. 2, pp. 129-151.
FAO (2010), “FAOSTAT: food and agriculture organization of the United Nations”, available at: http://faostat3.fao.org (accessed 24 January 2018).
Fitzpatrick, M.C., Gove, A.D., Sander, N.J. and Dunn, R.R. (2008), “Climate change, plant migration, and range collapse in a global biodiversity hotspot: the Banksia (Proteaceae) of Western Australia”, Global Change Biology, Vol. 14 No. 6, pp. 1337-1352.
Florêt, C. and Pontanier, R. (1982), “L’aridité en Tunisie présaharienne: climat, sol, végétation et aménagement”, Travaux et document de l’ORSTOM n° 150, Orstom editions, Paris.
Freier, K.P., Schneider, U.A. and Finckh, M. (2011), “Dynamic interactions between vegetation and land use in semi-arid Morocco: using a Markov process for modeling rangelands under climate change”, Agriculture, Ecosystems and Environment, Vol. 140, pp. 462-472.
Fuhlendorf, S.D. and Engle, D.M. (2001), “Restoring heterogeneity on rangelands: ecosystem management based on evolutionary grazing patterns: we propose a paradigm that enhances heterogeneity instead of homogeneity to promote biological diversity and wildlife habitat on rangelands grazed by livestock”, BioScience, Vol. 51 No. 8, pp. 625-632.
Guisan, A. and Theurillat, J.P. (2000), “Assessing alpine plant vulnerability to climate change: a modeling perspective”, Integrated Assessment, Vol. 1 No. 4, pp. 307-320.
Guisan, A. and Zimmermann, N.E. (2000), “Predictive habitat distribution models in ecology”, Ecological Modelling, Vol. 135 Nos 2/3, pp. 147-186.
Guma, I.R., Padrón-Mederos, M.A., Santos-Guerra, A. and Reyes-Betancort, J.A. (2010), “Effect of temperature and salinity on germination of Salsola vermiculata L. (Chenopodiaceae) from Canary Islands”, Journal of Arid Environments, Vol. 74 No. 6, pp. 708-711.
Hernandez, P.A., Graham, C.H., Master, L.L. and Albert, D.L. (2006), “The effect of sample size and species characteristics on performance of different species distribution modeling methods”, Ecography, Vol. 29 No. 5, pp. 773-785.
Hijmans, R.J. and Graham, C.H. (2006), “The ability of climate envelope models to predict the effect of climate change on species distributions”, Global Change Biology, Vol. 12 No. 12, pp. 2272-2281.
Hijmans, R.J., Cameron, S.E., Parra, J.L., Jones, P.G. and Jarvis, A. (2005), “Very high resolution interpolated climate surfaces for global land areas”, International Journal of Climatology, Vol. 25 No. 15, pp. 1965-1978.
Holochek, J.L., Pieper, R.D. and Herbel, C.H. (2004), Range Management Principles and Practices, Pearson Prentice Hall, Upper Saddle River, NJ.
Huang, Z., Zhang, X., Zheng, G. and Gutterman, Y. (2003), “Influence of light, temperature, salinity and storage on seed germination of Haloxylon ammodendron”, Journal of Arid Environments, Vol. 55 No. 3, pp. 453-464.
Hudson, L.N., Newbold, T., Contu, S., et al. (2014), “The PREDICTS database: a global database of how local terrestrial biodiversity responds to human impacts”, Ecology and Evolution, Vol. 4 No. 24, pp. 4701-4735.
Intergovernmental Panel on Climate Change (IPCC) (2000), Summary for Policymakers. Emissions Scenarios. A Special Report of IPCC Working Group III, Cambridge University Press, Cambridge.
Intergovernmental Panel on Climate Change (IPCC) (2007), Climate Change 2007: The Physical Science Basis. Contribution of Working Group I to the Fourth Assessment Report of the Intergovernmental Panel on Climate Change, Cambridge University Press, Cambridge, and New York, NY.
Jochum, G.M., Mudge, K.W. and Thomas, R.B. (2007), “Elevated temperatures increase leaf senescence and root secondary metabolite concentrations in the understory herb Panax quinquefolius (Araliaceae)”, American Journal of Botany, Vol. 94 No. 5, pp. 819-826.
Le Houérou, H.N. (1980), “L’impact de l’homme et ses animaux sur la forêt méditerranéenne. Deuxième partie”, Forêt Méditerranéenne, Vol. 2 No. 1, pp. 31-44.
Le Houérou, H.N. (1996), “Use of fodder trees and shrubs (trubs) in the arid and semi-arid zones of West Asia and North Africa: history and perspectives”, in Gintzburger, G., Bounejmate, M. and Nefzaoui, A. (Eds), Fodder Shrub Development in Arid and Semiarid Zones, Vol. 1, International Center for Agricultural Research in the Dry Areas, Aleppo, pp. 9-53.
Lemaire, G., Franzluebbers, A., de Faccio, C.P.C. and Dedieu, B. (2014), “Integrated crop-livestock systems: strategies to achieve synergy between agricultural production and environmental quality”, Agriculture, Ecosystems and Environment, Vol. 190, pp. 4-8.
Louhaichi, M. and Tastad, A. (2010), “The Syrian steppe: past trends, current status and future priorities”, Rangelands, Vol. 32 No. 2, pp. 2-7.
Louhaichi, M., Clifton, K., Kassam, S. and Werner, J. (2016a), “Overlooked benefits and services of grasslands to support policy reform”, Options Méditerranéennes, Series A: Mediterranean, Vol. 114, pp. 301-312.
Louhaichi, M., Ghassali, F., Salkini, A.K. and Petersen, S.L. (2012), “Effect of livestock grazing on rangeland plant communities within Syrian landscape depressions”, Journal of Arid Environments, Vol. 79, pp. 101-106.
Louhaichi, M., Yigezu, A.Y., Werner, J., Dashtseren, L., El-Shater, T. and Ahmed, M. (2016b), “Financial incentives: possible options for sustainable rangeland management?”, Journal of Environmental Management, Vol. 180, pp. 493-503.
McKeon, G.M., Stone, G.S., Syktus, J.I., Carter, J.O., Flood, N.R., Ahrens, D.G., Bruget, D.N., Chilcott, C.R., Cobon, D.H., Cowley, R.A., Crimp, S.J., Fraser, G.W., Howden, S.M., Johnston, P.W., Ryan, J.G., Stokes, C.J. and Day, K.A. (2009), “Climate change impacts on Northern Australian rangeland livestock carrying capacity: a review of issues”, The Rangeland Journal, Vol. 31 No. 1, pp. 1-29.
Metzger, M.J., Rounsevell, M.D.A., Acosta-Michlik, L., Leemans, R. and Schröter, D. (2006), “The vulnerability of ecosystem services to land use change”, Agriculture, Ecosystems and Environment, Vol. 114, pp. 69-85.
Millar, C.I., Stephenson, N.L. and Stephens, S.L. (2007), “Climate change and forests of the future: managing in the face of uncertainty”, Ecological Applications, Vol. 17 No. 8, pp. 2145-2151.
Murad, N. (1996), “Native shrubs in sheep diets”, in Gintzburger, G., Bounejmate, M., Nefzaoui, A., (Eds), Fodder Shrub Development in Arid and Semiarid Zones, Vol. 1, International Center for Agricultural Research in the Dry Areas, Aleppo, pp. 109-121.
Nefzaoui, A., El Mourid, M. and Louhaichi, M. (2014), “The tribe – platform of participatory local development and management of communal rangeland resources”, Journal of Arid Land Studies, Vol. 24 No. 1, pp. 57-60.
Nordblom, T. (1992), “Characterization of year-round sheep feed and grazing calendar of Bedouin flocks in the Northwestern Syrian steppe”, ICARDA annual report, Aleppo.
O’Brien, K., Leichenko, R., Kelkar, U., Venema, H., Aandahl, G., Tompkins, H., Javed, A., Bhadwal, S., Barg, S., Nygaard, L. and West, J. (2004), “Mapping vulnerability to multiple stressors: climate change and globalization in India”, Global Environmental Change, Vol. 14, pp. 303-313.
Ouled Belgacem, A. and Louhaichi, M. (2013), “The vulnerability of native rangeland plant species to global climate change in the West Asia and North African regions”, Climatic Change, Vol. 119 No. 2, pp. 451-463.
Pearson, R.G. and Dawson, T.P. (2003), “Predicting the impacts of climate change on the distribution of species: are bioclimate envelope models useful?”, Global Ecology and Biogeography, Vol. 12 No. 5, pp. 361-371.
Petersen, S.L., Stringham, T.K. and Roundy, B.A. (2009), “A process-based application of state-and-transition models: a case study of Western Juniper (Juniperus occidentalis) encroachment”, Rangeland Ecology and Management, Vol. 62, pp. 186-192.
Peterson, A.T., Papes, M. and Eaton, M. (2007), “Transferability and model evaluation in ecological niche modeling: a comparison of GARP and MaxEnt”, Ecography, Vol. 30, pp. 550-560.
Peterson, A.T., Soberón, J., Pearson, R.G., Anderson, R.P., Martínez-Meyer, E., Nakamura, M. and Araújo, M.B. (2011), Ecological Niches and Geographic Distributions. Monographs in Population Biology, Princeton University Press, Princeton, NJ.
Phillips, S.J., Anderson, R.P. and Schapire, R.E. (2006), “Maximum entropy modeling of species geographic distributions”, Ecological Modelling, Vol. 190 Nos 3/4, pp. 231-259.
Post, G.E. and Dinsmore, J.E. (1933), Flora of Syria, Palestine and Sinai. A Handbook of the Flowering Plants and Ferns, Native and Naturalized from the Taurus to Ras Muhammad and Form the Mediterranean Sea to the Syrian Desert, Vol. 2, American Press, Beirut.
Quiroga, R.E., Golluscio, R.A., Blanco, L.J. and Fernández, R.J. (2010), “Aridity and grazing as convergent selective forces: an experiment with an arid Chaco bunchgrass”, Ecological Applications, Vol. 20 No. 7, pp. 1876-1889.
Rebelo, H. and Jones, R. (2010), “Ground validation of presence-only modelling with rare species: a case study on barbastelles Barbastella barbastellus (Chiroptera: Vespertilionidae)”, Journal of Applied Ecology, Vol. 47 No. 2, pp. 410-420.
Reitsma, K.D., Dunn, B.H., Mishra, U., Clay, S.A., DeSutter, T. and Clay, D.E. (2015), “Land-use change impact on soil sustainability in a climate and vegetation transition zone”, Agronomy Journal, Vol. 107 No. 6, pp. 2363-2370.
Salama, F.M., Abd El-Ghani, M.M., El Naggar, S.M. and Baayo, K.A. (2005), “Vegetation structure and environmental gradients in the Sallum area, Western Mediterranean Coast, Egypt”, Ecologia Mediterranea, Vol. 31 No. 1, pp. 15-32.
Schneider, R., Boutin, S., Stelfox, J. and Wasel, S. (2007), “Scenarios are plausible stories about the future, not forecasts”, Ecology and Society, Vol. 12 No. 1, pp. r4.
Shcheglovitova, M. and Anderson, R.P. (2013), “Estimating optimal complexity for ecological niche models: a jackknife approach for species with small sample sizes”, Ecological Modelling, Vol. 269, pp. 9-17.
Stringham, T.K., Krueger, W.C. and Shaver, P.L. (2003), “State and transition modeling: an ecological process approach”, Journal of Range Management, Vol. 56 No. 2, pp. 106-113.
Thornton, P.K., van de Steeg, J., Notenbaert, A. and Herrero, M. (2009), “The impacts of climate change on livestock and livestock systems in developing countries: a review of what we know and what we need to know”, Agricultural Systems, Vol. 101 No. 3, pp. 113-127.
Acknowledgements
This work was supported by the International Center for Agricultural research in the Dry Areas (ICARDA) and the CGIAR Research Program on Livestock (CRP Livestock). The authors thank several anonymous reviewers for their critical review. The opinions expressed here belong to the authors and do not necessarily reflect those of CRP Livestock, ICARDA or CGIAR.