Abstract
Purpose
The paper seeks to bridge the already familiar benefits of 3D printing (3DP) to the rehabilitation of cultural heritage, still based on the use of complex and expensive handcrafted techniques and scarce materials.
Design/methodology/approach
A compilation of different information on frequent anomalies in cultural heritage buildings and commonly used materials is conducted; subsequently, some innovative techniques used in the construction sector (3DP and 3D scanning) are addressed, as well as some case studies related to the rehabilitation of cultural heritage building elements, leading to a reflection on the opportunities and challenges of this application within these types of buildings.
Findings
The compilation of information summarised in the paper provided a clear reflection on the great potential of 3DP for cultural heritage rehabilitation, requiring the development of new mixtures (lime mortars, for example) compatible with the existing surface and, eventually, incorporating some residues that may improve interesting properties; the design of different extruders, compatible with the new mixtures developed and the articulation of 3D printers with the available mapping tools (photogrammetry and laser scanning) to reproduce the component as accurately as possible.
Originality/value
This paper sets the path for a new application of 3DP in construction, namely in the field of cultural heritage rehabilitation, by identifying some key opportunities, challenges and for designing the process flow associated with the different technologies involved.
Keywords
Citation
Jesus, M., Guimarães, A.S., Rangel, B. and Lino Alves, J. (2023), "The potential of 3D printing in building pathology: rehabilitation of cultural heritage", International Journal of Building Pathology and Adaptation, Vol. 41 No. 3, pp. 647-674. https://doi.org/10.1108/IJBPA-03-2022-0053
Publisher
:Emerald Publishing Limited
Copyright © 2023, Manuel Jesus, Ana Sofia Guimarães, Bárbara Rangel and Jorge Lino Alves
License
Published by Emerald Publishing Limited. This article is published under the Creative Commons Attribution (CC BY 4.0) licence. Anyone may reproduce, distribute, translate and create derivative works of this article (for both commercial and non-commercial purposes), subject to full attribution to the original publication and authors. The full terms of this licence may be seen at http://creativecommons.org/licences/by/4.0/legalcode
1. Introduction
The Construction industry is not only known for being one of the most pollutant and resource-consuming ones, but also for its lack of technological progress from some time now.
Industry 4.0 (Fourth Industrial Revolution) intends to bond manufacturing systems with information and communication technologies (ICTs), severely disrupting the current industry structure and business models (Craveiro et al., 2019; Dalenogare et al., 2018). In the construction sector, this change is often termed as Construction 4.0, including ground-breaking concepts, such as digitalisation and automation, mostly driven by shortage of skilled manpower, productivity gains and environmental benefits (Craveiro et al., 2019). The digitalisation has been firmly established in the sector through the continuous use of computer aided design (CAD) software in the design phase and, more recently, with the use of Digital Twins and Building Information Modelling (BIM) capable of synthesising all the information of a building throughout its lifecycle (García et al., 2017). Automation in Construction seeks to further revolutionise the sector by implementing faster, less costly and more material-efficient production processes. In this context, a renewed interest in off-site construction and pre-fabrication has been witnessed with several projects, where building elements are being produced off-site and assembled in situ (FIEC, 2017). Other innovative construction methods are now being explored to maximise the benefits of off-site construction, prefabrication and digitalisation, where 3D printing (3DP) technologies are included among the most promising ones.
There are several reasons that motivate the construction sector towards digital fabrication, in particular production time, cost reduction and architectural freedom (Lim et al., 2012). Other well-known benefits include material consumption and waste reduction, printing equipment autonomy and continuous manufacturing, flexibility to print both in situ and off-site elements, modules or entire structures and the absence of formworks of critical importance when using concrete-like materials (Wolfs and Salet, 2016; Yossef and Chen, 2015). However, given the unique and conservative nature of the sector, the gradual adoption of digital technologies occurred as supportive tools for traditional and well-established methods, and 3DP in construction has been limited to new-residential projects, mostly experimental or dedicated to affordable housing.
According to UNESCO (2009), cultural heritage contemplates “artefacts, monuments, a group of buildings and sites, museums that have a diversity of values including symbolic, historic, artistic, aesthetic, ethnological or anthropological, scientific and social significance”.
The rehabilitation of this type of buildings is seen as a sensitive area, due to the complexity, diversity and costs involved for a harmonious and compatible effort with the initial structure. Currently, several techniques have been studied to protect the historical and cultural heritage in a more sustainable, economic and efficient way (Liudmila and Balkiz, 2019). To date, printing entire buildings is still challenging, not only due to economic reasons and market acceptance, but also due to the lack of suitable construction codes and guidelines. Therefore, the construction industry is eager to explore new business models and market segments like rehabilitation and retrofitting and respond to the growing demand for customisable building elements. Thus, this technology is certainly capable of producing small components to replace obsolete and unavailable ones in, for instance, heritage buildings, since they require specific fittings and rich ornamentation (FIEC, 2017).
This paper seeks to introduce the concept of 3DP, by identifying significant benefits of its use, as an alternative to the current manual construction processes; a brief overview of cultural heritage rehabilitation and an analysis of the current challenges faced by historical buildings, in particular the difficulties associated to building pathologies; and a discussion of 3DP's potential in bridging some of the constraints currently experienced in this field, supported by ongoing case studies.
2. Cultural heritage rehabilitation
2.1 Framework
When talking about built heritage and rehabilitation, some uncertainties about the terminology to be applied often arise, being not only important to understand and clearly distinguish each one, but also the tasks involved in carrying them out. Below, it is possible to understand the definitions and actions inherent to the terminology frequently used, according to EN 15898:2011 (CEN, 2011).
Conservation – Measures and actions aimed at safeguarding cultural heritage, while respecting its significance, including its accessibility to present and future generations. There are three types of conservation:
Preventive – Measures and actions aimed at avoiding or minimising future damage, deterioration and loss and, consequently, any invasive intervention;
Remedial – Actions applied directly to an object to arrest deterioration and/or to limit damage;
Restoration – Actions applied to a stable or stabilised object aimed at facilitating its appreciation, understanding and/or use, while respecting its significance and the materials and techniques used.
Maintenance – Periodic preventive conservation actions aimed at sustaining an object in an appropriate condition to retain its significance;
Rehabilitation – Interventions on an immovable object in order to recover an inferred earlier functionality, to adapt it to a different function or to standards of comfort, safety and access;
Reconstruction – Re-establishment of an object to an inferred earlier form using existing or replacement material;
Repair – Actions applied to an object or part of it to recover its functionality and/or its appearance;
Reintegration – Addition of material in order to facilitate the perception and understanding of an object, e.g. retouching, gap filling, insertion, in-painting, etc.
Renovation – Action of renewing an object without necessarily respecting its material or significance.
In this kind of building, it is crucial to conduct periodic inspections and plan appropriate maintenance actions, in order to prevent the appearance of anomalies and consequent degradation of different building elements.
The major difficulties associated to building rehabilitation are related to the improvement of functional and constructive performance standards, based on quality parameters established in the legislation; for historic buildings, additional difficulties emerge, related to the use of techniques compatible with traditional construction systems (Appleton, 2003).
Rehabilitation of historic buildings is essential for the preservation of their historical, cultural and architectural value, and it is motivated by one (or combined) number of factors, namely (CIB, 2010).
Appearance of visible defects;
Damage after a particular event capable of affecting its stability (earthquakes, tsunamis, etc.);
The changes of the use of the building to more severe conditions;
Imposition of new codes whenever an increase of acting loads (earthquake action, traffic action, etc.) is observed.
Whenever it is possible, the rehabilitation works on built heritage should follow key principles, according to the Venice Chart (1964) (CIB, 2010).
Structural stability assurance;
Cultural value respect;
Minimum intervention;
Reversibility of the intervention;
Integration on the existing building;
Compatibility of the materials;
Minimum cost.
2.2 Building materials and elements
Historic buildings are known for their peculiar character and beauty and are still a source of inspiration for new construction. The simple fact that they are resilient structures that have survived the passage of time, built at a time when techniques were limited and materials were apparently poor, can impress any party involved in the constructive process.
Much of this success is due to the careful selection and design of different building materials, which, at the time, were mainly extracted in the vicinity of the construction site, not only due to transport restrictions, but also to their immediate availability with no apparent cost (Wines and Jodidio, 2000).
A great part of historic buildings present characteristics of resistant masonry, with their structure being composed of load-bearing walls, columns, buttresses, vaults and domes (Döndüren and Sişik, 2017). As for the supporting materials, the selection fell on a wide variety of stones (red, granite, limestone and sandstone), adobe and, for the joints, lime mortar (SAHC, 2019 citing M. Kilumine). Other materials, such as timber, steel (iron), glass and ceramic tiles were also highly used.
However, as mentioned above, old materials, regardless of their qualities, gradually become eroded and deteriorate, requiring repair or replacement. In the next chapter, it will be possible to address the anomalies (and causes) that contribute to a faster degradation of materials in different cultural building elements.
2.3 Anomalies and general treatment procedures
Since cultural heritage buildings comprise a significant economic opportunity and carry a characteristic socio-cultural legacy of the place they belong to, they deserve greater preservation to maintain their functional and safety levels. Besides the expected ageing and degradation of the materials (or even their compatibility), other factors contribute to the development of anomalies in historic buildings, as summarised above (Prieto et al., 2017; Kumar et al., 2020).
Design and execution errors – Poor qualification of manpower, inappropriate selection of materials or incompatibility between them, lack of conformity to design, etc.
Environmental actions – Incident rainfall, wind, solar radiation and air pollution (all of these factors are dependent on the geographical location of the building, affected by altitude, proximity to coastal areas and sun exposure);
External mechanical actions – Stress concentration, differential settlements, shrinkage/expansion of elements, etc.
Unusual events – War conflicts, earthquakes, fires and floods;
Use and maintenance – Lack of conservation and proper maintenance actions, lack of periodic inspections, inadequate ventilation of inner rooms, etc.
Some examples of frequent anomalies and respective causes are listed in Table 1.
The main issue associated to the treatment of anomalies is the complexity in understanding their cause(s), which severely limits the interventions to be carried out. For instance, loss of adherence, observed in Table 1, may be due to the choice of materials (quality and compatibility), lack of support preparation or even the presence of damp, making it difficult to define an appropriate diagnosis and to choose the type of solution to adopt in advance.
The repair and rehabilitation processes are fairly extensive, involving several stages: identification of the anomaly; data collection and analysis; elaboration of the diagnosis, with selection of the most appropriate technique(s); viability assessment of the technique(s); execution of intervention; monitoring of the intervention performance.
The starting point is based on a complete inspection of the building, evaluating the conservation conditions, the resistance of the structural elements, the materials used and a survey and characterisation of the existing anomalies. This work is essential to achieve a harmonisation between the historical and cultural value of the former space and the contemporaneous intervention, which must respect ancient elements (Tavares et al., 2011).
Regarding data collection, diagnosis tests can be in situ, through non-destructive, semi-destructive or destructive methods. However, accessibility problems and eventual destruction of certain elements for sample extraction associated to heritage buildings only allow the use of non-destructive techniques. With the current development of sensors and analysis software, it is possible to accurately characterise materials, detect wear and degradation and study compatible materials (Moropoulou et al., 2013).
It is also important to mention that the adopted solutions should rely as much as possible on traditional construction techniques, with solutions that are little intrusive and quite reversible, to ensure the initial characteristics of the space and with no uncharacteristic construction elements (Tavares et al., 2011).
After the execution of the works, necessarily conducted by highly qualified professionals, it will be crucial to introduce regular maintenance policies to guarantee the quality of the intervention and the correct conservation of the building.
3. 3D Printing in construction
3.1 Framework
According to ISO/ASTM 52900:2015 (ISO/ASTM, 2015), additive manufacturing (AM) is the “process of joining materials to make parts from 3D model data, usually layer upon layer, as opposed to subtractive manufacturing and formative manufacturing methodologies”. AM includes seven different process categories (ASTM International, 2013).
Binder Jetting – A liquid bonding agent is dropped to join powder materials;
Directed Energy Deposition – Focused thermal energy (e.g. laser, electron beam) is used to fuse deposited materials by melting them;
Material Extrusion – A nozzle or orifice continuously pours fresh materials, e.g. Fusion Deposition Modelling (FDM);
Material Jetting – Droplets of build material are selectively deposited;
Powder Bed Fusion – Thermal energy fuses certain regions of a powder bed, e.g. Selective Laser Sintering (SLS);
Sheet Lamination – Sheets of material are glued to form an object;
Vat Photopolymerisation – A liquid photopolymer in a vat is selectively cured by a light-activated polymerisation, e. g. Stereolithography (SLA).
In Construction, this technology is being studied for the last twenty years by different groups of researchers worldwide (Figure 1). Techniques such as Contour Crafting and Concrete Printing (extrusion based techniques that use high-performance cement mortars) or D-Shape (binder jetting technique) created a new thread for the development of building and architectural components in a large scale (Lim et al., 2011). Its potential was demonstrated through the immediate conception of different kinds of structures, such as modular components (bricks and walls), whole houses, bridges or pieces of art and decoration.
3.2 Material extrusion techniques
In Construction, FDM technique was the inspiration for the development of several printing equipment, as will be seen below. The extruded material must fulfil some requirements to support its own weight and the load of each subsequent layer without any deformation during the process. In the next sections (3.2.1; 3.2.2; 3.2.3), some noteworthy examples of material extrusion techniques will be described.
3.2.1 Gantry systems
Contour Crafting (CC) is an American company created by Dr. Behrokh Khoshnevis in 2004 with the aim of improving the optimisation in construction with cement-based materials, focusing on the rapid conception of houses worldwide with reduced costs and waste production. This automatic construction process is based on extruding a cement-based material, where the gantry system makes the printing nozzle moving along X and Z directions, while two parallel sliding structures enable the nozzle's movement along Y direction (Figure 2). The extrusion method is hybrid: the outside edges (rims) are built first, followed by the filling of the area between the opposite rims with a filler material (such as concrete). During the extrusion phase, two trowels attached to the printing nozzle enable the creation of smooth and accurate surfaces (Khoshnevis, 2004).
Concrete Printing is another largescale printing technique similar to Contour Crafting, built by researchers at Loughborough University, United Kingdom, with the aim of producing concrete structures without labour-intensive formwork (Plate 1) (Lim et al., 2011). This technique is based on the extrusion of a cement mortar, in a layer-by-layer process, where the printing head is mounted on an overhead crane and can freely move in X, Y and Z directions. Here, there is only one deposition nozzle, with approximately 9 mm of diameter, that continuously deposits fresh concrete layers (6 mm thick) that are smoothly transported from a delivery pipe with the help of a pump (Ma et al., 2017).
One of the first prototype printing systems included a print bed of 5.4 × 4.4 × 5.4 m (L × W × H) and was tested with cement and gypsum-based materials. More recently, a research team at Eindhoven University of Technology (TU/e), Netherlands, presented their huge concrete 3D printer with a four-axis gantry robot and a printing bed of 9 × 4.5 × 3 m (Ma et al., 2017).
3.2.2 Robotic systems
CyBe, a Netherlands-based company established in 2013, is working on the conception and development of concrete 3D printers since its beginning. The so-called “robotic 3D printers” comprise a concrete extruder mounted on a robotic arm capable of moving in six axes (Plate 2). Even though they cannot print large elements, like gantry systems, their additional rotation axes allow greater freedom and detail in the creation of elements. Additionally, these systems are compatible with several printheads, enabling printing speeds in the range 10 mm/sec - 400 mm/sec (3DPrint.com, 2015b).
3.2.3 Crane systems
Apis Cor came up with a rotative construction crane that enables a printing area of 132 m2 with the goal of developing a less cumbersome and easy-transportable 3D printer (only requires a truck). This printing robot can be easily disassembled, installed (only requires 30 min) and transported (3DPrint.com, 2015a).
3.3 Binder jetting techniques
Binder Jetting is also one of the main types of AM processes. This technique considers the deposition of thin layers of powder material (metals, sand, or ceramics in a granular form) over a powder bed that will later be bonded by the binder material. After the 3D object is completed, the unbound material that remained inside is removed with a vacuum cleaner, which can be recycled for another 3DP project, resulting in little or no waste (Tay et al., 2017).
3.3.1 D-shape
One of the main large-scale Binder Jetting printers is the D-Shape. This technology was created in 2007 by the Civil Engineer Enrico Dini and shows up as a portal structure with four columns where one gantry distributes and spreads granular material and the other one holds a multi-binder-jetting head that uses an aqueous solution of different additives (Dini developed a special inorganic binder that is cheap, ecologic and has low viscosity, being capable of giving structural strength to the mixture) (Plate 3). The granular material is usually a “cementitious micro-concrete material made by particles of various materials and granulometries”, such as crushed stones, recycled materials, crush-recycled materials (glass, demolition rejects, chipped wood, or plastic, etc.) and expanded clay aggregates (Dini, 2020).
This process has been used in several projects, including “Radiolaria” (Plate 4), an 1.60 m tall piece and a pedestrian bridge located in Madrid.
3.4 3D printing materials
Materials used for 3DP in Construction are normally based on mortars with cementitious binders (Plate 5), given the pre-existing knowledge about them and their attractive physical and mechanical properties (section 3.4.1). In parallel, new mixtures based on Earth/local materials (section 3.4.2) have been tested, due to its lower environmental impact when compared to cement-based ones (Perrot et al., 2018; Alhumayani et al., 2020).
3.4.1 Cement-based materials
Designing cementitious mortars for 3DP is not trivial, as good workability, printability, and extrudability must be guaranteed to pour a continuous filament of material through the printing nozzle (Ma et al., 2017). Static yield stress and plastic viscosity must also be substantial to prevent segregation, filament deformation and buckling failure. To control printability and buildability, a high dosage of cement is typically used in concrete/mortar for 3DP (Rushing et al., 2019). The most important properties are described in Table 2.
Cementitious mortars for 3DP have been studied for quite a long time by many entities worldwide. However, little is known about the composition of their mortars, due to confidentiality agreements with the raw material manufacturers. Table 3 collects some compositions found in the literature.
In short, the most used components in 3D printable cementitious mortars were synthesised by Craveiro et al. (2019), as seen in Table 4.
3.4.2 Earth-based materials
Mud constructions have been used since pre-historic times, offering important benefits like fire protection, stiffness, great strength, winter heating and summer cooling, due to their good thermal inertia properties. Furthermore, clay, a material typically used in vernacular architecture, can absorb and restore water, which is ideal to control environmental moisture, contributing for a healthy indoor climate (IAAC, 2016). However, regarding 3DP, the main issue with these materials lies in the complexion of conceiving a mixture that allows, simultaneously, fast casting and sufficient strength in the dry state (Perrot et al., 2018).
In 2016, Institute for Advanced Architecture of Catalonia (IAAC) started the development of a project related with the use of Earth/soil materials that would incorporate a climatic performative design. With a new mixture (based clay, sand and water), 3D printed parts were produced to explore the optimisation of the design, ensuring maximum performance concerning solar radiation, wind and structural behaviour, having several radiuses of self-shading that enable the optimisation of the east and west sun. Moreover, the incorporation of different types of openings was thought to maximise the natural daylight potential, taking advantage of wind to increase the thermal insulation of the structure (IAAC, 2016).
WASP developed a small building (EREMO) using only local materials (Plate 6). During the design phase, some considerations were addressed, namely thermal and acoustic insulation of the walls and an earthquake-proof configuration. The mixture included dirt, water and straw. Also, the addition of sand and lime enabled the walls to maintain their natural composition and be more breathable (WASP, 2017).
4. The technological side of building rehabilitation: opportunities and challenges
4.1 Framework
With the appearance of materials such as steel and concrete, there was a significant architectural change in new constructions, which were more directed towards efficiency and utility, abandoning the former ornamentation. As the materials used in the past fell into disuse and skilled labour was drastically reduced, it became increasingly difficult to consider the rehabilitation of buildings with heritage value.
In addition, the reconstruction of parts or the replacement of elements that no longer perform their function is a complex task for two reasons.
Dealing with very ancient buildings, the insufficiency or inexistence of drawings and the rest of the project documentation hinders rehabilitation works (Lourenço, 2002; Moropoulou et al., 2013); to address this constraint, the solutions used until then involved visual observation or manual mapping, considered cumbersome and imprecise (Armesto-González et al., 2010);
A considerable proportion of historic buildings are built of stone and masonry and there are often elements with detailed finishes, such as complex curves (Armesto-González et al., 2010). The reproduction of these elements is complex and expensive and is normally carried out by specialised technicians, who are increasingly rare.
However, 3DPC can play a major role in maintenance, repair and retrofit of buildings and infrastructures. Digital mapping technologies, such as laser scanning and photogrammetry, are capable of extracting precise and complete geometrical information of buildings and construction elements, highlighting deteriorated areas. In association with 3DPC, these technologies can amplify their immense potential in cultural heritage rehabilitation sector by allowing to reproduce damaged elements of historical/ornamental value.
4.2 3D scanning, 3D digital representation and physical reproduction of replicas
Photogrammetry reconstructs 3D spaces through a set of high-resolution photographs, captured by conventional cameras or, more recently, drones (El-Hakim et al., 2007). It is, therefore, a non-invasive and contactless method, avoiding the risk of accidental damage, which could compromise the durability of the building. Another fundamental characteristic of photogrammetry is the availability of low-cost equipment, composed of cameras (without major specific requirements) and other simpler elements, such as tripods, tablets, lasers (to measure distances), measuring tape or crest (Wingtra, 2021).
In turn, laser scanning is based on fast-automatic acquisition of spatial data of existing buildings or complex surfaces by millions of points per second with high accuracy (millimetre to metre) (Leica Geosystems, 2016; Lagüela et al., 2014; Fröhlich and Mettenleiter, 2004). This technology is quite suitable for cultural heritage, since it provides the monitoring of interventions, the detection of defects and the reproduction of existing models. Unlike photogrammetry, it requires expensive equipment, qualified professionals and a correct planning of the scanner stations, since there may be elements blocking its range (Bhatla et al., 2012).
However, both techniques allow the creation of point clouds that can be later converted into high resolution 3D G-code models of geometric shapes and objects.
The potential of 3DP for the rehabilitation of buildings/elements with heritage value is only perceptible through the visualisation and demonstration of actual practical examples. Nevertheless, the clear lack of academic papers within the scope of this study certainly does not detract from its relevance to the industry; indeed, the contribution of each becomes more valuable and leaves more room for future developments.
For a better understanding of the aim of this article, namely the benefits brought by the application of 3DP to the cultural heritage rehabilitation sector, the literature review conducted tries to tackle two distinct aspects.
In situ, non-horizontal extrusion of material onto surfaces, as observed in Plates 11 and 12;
Replication of elements/parts of historical buildings capable of being later assembled on site, as is the case of the remaining examples presented in chapter 4.2.
It was also observed, in some of the cases, that 3DP was used for the manufacture of moulds with complex geometries (Plate 11 and Figure 3), crucial to produce the replicas, finding it pertinent to include those cases. Also, not all cases employed material extrusion techniques (chapter 3.2); since a high level of precision was sometimes required, binder jetting techniques were the most convenient in that regard, as seen in the voxeljet example.
Xu et al. (2017) pioneered this research field combining 3D scanning with 3DP for the refurbishment of historic structures and ornamental elements. The authors restored the plinth of a historic stone column (Plate 7), which is essential to sustain higher loads, ensure lateral support and prevent water deterioration, while providing a more aesthetic finish to the column.
This task of digital reproduction and consequent reconstruction of a component of a historic building entails several steps, from the 3D scanning of the zone to be interventioned to the extrusion of the desired material, which are (Xu et al., 2017).
Data acquisition, through the 3D scanner, and obtention of the point cloud data (PCD), to be included later in a 3D digital model;
Creation of the digital file, where a STL file (for decomposing the digital part into layers) is produced from the 3D digital model, which will also give information about the path of the extrusion nozzle, as previously explained;
Printing of the piece, through the algorithms created in the previous step, responsible for the movement of the printer and the extrusion process;
Installation of the component in the damaged zone, with a margin for post-processing (polishing, for example).
Using a cementitious mortar, it was possible to obtain a similar performance to the original stone in terms of physical properties (Plate 8).
With a similar approach, Yeon et al. (2018), via 3DP, conceived a concrete patch to repair a spall damage of a road. In these cases, pouring Portland cement concrete directly over the damaged area is not advisable, due to the normal occurrence of shrinkage during the curing process.
At first, as expected, a 3D computer model of the damaged area was created, using photogrammetry (several pictures were taken from various locations). After that data collection, the 3D computer model of the spall damage's surface was attainable with Autodesk ReMake, followed by the preparation of the respective 3D model for printing the plastic formwork, as seen in Plate 9 (left). Then, fresh concrete was placed on it and was cured in a room with a controlled temperature and relative humidity for 28 days, to avoid the abovementioned shrinkage (the authors mentioned that it was effective). At last, a specific adhesive was applied in the damaged area to properly attach the concrete patch, preventing its detachment due to external forces (Plate 9 (right)) (Yeon et al., 2018).
A collaboration between archaeologists from Oxford University's Institute for Digital Archaeology (IDA) and United Nations Educational, Scientific and Cultural Organization (UNESCO) resulted in the recreation of Palmyra's Arch of Triumph, 1800-year-old Roman arch from Syria, razed by the extremist group ISIS. The prototype was firstly erected in London's Trafalgar Square, in 2016, and has about 2/3 (6 m tall) of the scale of the original, weighing 11 tonnes and produced via 3DP technology, based on 2D pictures of the original arch. Two RobotMills robots developed different blocks, later assembled onsite (Alexandrea, 2017, Jalabi, 2016).
Despite the intention of reconstructing the original monument, some questions and criticisms were raised about IDA, related to Khunti (2018) and Factum Foundation (2016).
Honesty – The decontextualisation of the arch caused by the lack of communication reduced the significance of its reproduction as a symbol of solidarity with the Syrian heritage and the human loss provoked;
Authenticity – IDA used photographs from tourists to reconstruct the arch, resulting in clear inaccuracies from the use of photogrammetry (Factum Foundation compared the lack of detailed employed in the 3D printed arch to the original one); also, the use of other stone (marble quarried in Italy) was not appropriate, given its colour and texture, and the size of the structure (2/3 of the original) largely contributed to the lack of authenticity in the reproduction;
Accessibility – The arch was exhibited in London, New York and Dubai, locations not accessible for a significant part of Syrian people, and the language distanced them from their own heritage; in addition, the restriction of online information about it (3D models and images) denied their access to it;
Ethical implications – Apart from the possibility of this reconstruction bringing another act of terror, it may have potentiated a wave of rapid re-building of historical heritage, without considering all the associated technical challenges and ethical implications; also, UNESCO has guidelines to ensure that reconstructions of this type of buildings must remain authentic, otherwise it will betray all the involved parties by the loss of significance.
3DION 3D network and voxeljet gathered efforts to produce replicas for the patrons of the Michael portal of the Cologne Cathedral. The material used by voxeljet provides a similar haptic experience of sandstone, a crucial factor in the choice of this company, also known for producing sand moulds and plastic models for worldwide industries (voxeljet, 2020).
At first, the patrons were scanned with Steinbichler 3D and Artec Spider scanners, the latter used for the flaws on the plaster models. Then, the acquired data was processed with the help of 3D Scanworks. The printing of 108 replicas took only 31 h, using the VX1000 3D printer and PMMA powder (polymethyl methacrylate, a synthetic, transparent thermoplastic), since it provided the tactile experience of sandstone. At last, the replicas were cleaned, infiltrated and impregnated with epoxy resin (voxeljet, 2020).
Another great example of a heritage building retrofit was conducted in Ferrara, Italy, more precisely at the grand Spada Palace, built in the 1800s. To respond to the owners' request (suggested by a specialist restoration engineer), Sismaitalia, a pioneer in visual communication, interior design and digital printing and the first Italian business to acquire a Massivit 1800 3D printer, was responsible to produce a cost-effective and temporary replacement for the Palace capitals (MASSIVit 3D, 2018).
The five capitals were printed in polystyrene in two sizes: a set of two with 48 cm high x 43 cm long x 21.5 cm thick and a larger set of three measuring 79 cm high x 79 cm long x 21.5 cm thick. Given the dual printhead of the 3D printer, a pair of capitals could be conceived in just 20 h. After filled, the capitals received a plaster finish and were painted with the same colour as the original parts, to guarantee a genuine aspect (MASSIVit 3D, 2018).
Federica Tisato claimed that with the abovementioned printer it is possible to “quickly produce the hollow structure and then strengthen it with polyurethane foam, in order to withstand the weight of the balcony”, ensuring that the manufacturing costs were very low (MASSIVit 3D, 2018).
Given the incidents that took place on the Notre-Dame Cathedral in 2019, several entities began to taught that 3DP could be a plausible solution to refurbish the building in the short five-year timeframe imposed by French president Emmanuel Macron (by 2024). If traditional methods of construction would be preferred, the reconstruction would certainly be delayed, since it is almost impossible to obtain the materials used in the former building (Lutetian limestone was taken from mines that are now buried under the city and large oak beams used in the timer roof were conceived from trees felled in the 13th century) and there is a huge lack of experts with the specific know-how for specialised tasks, being necessary to recruit, at least, 400 craftsmen, between master stone-cutters, woodworkers, quarrymen, roofers, sculptors and others (France 24, 2019).
Concr3de, a Dutch company which suggested the application of 3DP for the restoration of the Notre-Dame Cathedral, replicated the iconic gargoyle (Plate 10) found on the cathedral's roof with a mixture of limestone and ash, similar materials to the ones found after the fire. Available scans of the gargoyle were found on the Internet and then printed with an Armadillo White small-sized 3D printer (30 × 30 × 30 cm) with a precision of 0.1 mm and capable of working with stone and stone-like materials, guaranteeing high accuracy in the creation of replicas for all types of elements (deezen, 2019).
Even though, at present, it is almost impossible to obtain the materials used in the former building, as stated above, Eric Geboers, co-founder of Concr3de, stated that it would be possible to use the ash, dust and damaged stones remnants in the nave of the cathedral, converting them into a 3D printable powder with a yellowish grey colour. Also, there is already a full 3D scan of the cathedral since 2000, thanks to Andrew Tallon, which would serve as a starting point for the first printing parts (France 24, 2019).
According to Concr3de, with 3DP, not only the labour shortage could be fixed in the production of the more intricate pieces, but also skilled stonemasons would be required to fix the printed parts into place (deezen, 2019).
In a different approach, edg, a New York architecture and engineering firm focused on technological design and restoration of buildings believes that the potential of 3D-printing in Construction relies on complex concrete mould-making instead of solid 3D-printed parts, not so durable or aesthetically pleasing (The Architect's Newspaper, 2018).
To study that possibility, a 1940 building with complex ornamentation that was about to be demolished served as a case study, where the company was responsible to restore and maintain the deteriorating ornamentation. After several studies of materials and mould thicknesses, edg's research team came up with a proper combination of material cost efficiency and strength (The Architect's Newspaper, 2018).
As seen in Figure 3, the final prototype, produced by voxeljet with voxeljet VX1000 printer and Sika concrete, is composed by a 3D printed form, a laser cut wire mesh and 3D printed stirrups, responsible for providing reinforcement for the case. Also, a plane connection system was designed to simplify the attachment to the original facade (The Architect's Newspaper, 2018).
4.3 Customisation of 3DP equipment and novel materials
Both gravity and the surrounding environment have always been conditional factors in AM processes, making it unviable to print parts on inclined surfaces or with different roughness. Given this situation, IAAC came up with an innovative extrusion equipment capable of neutralising the gravity effect during the printing process. Here, the created object results from the production of 3D curves instead of 2D successive layers, providing a greater complexity of the developed structures (IAAC, 2018).
In Spain, since 2014, HOLCIM have been studying and developing 3DP dry mortar solutions that use both cementitious materials and natural hydraulic lime. Aside from enabling significantly high printing speeds, these solutions are flexible and easily adaptable to any customer needs, since they can be printed vertically and directly onto walls, for ornamental purposes (Plate 11) (HOLCIM, 2020).
Similarly, other vertical extrusion techniques include the application of foam concrete (Lublasser et al., 2018) and cementitious materials with fly ash cenosphere (Bing et al., 2018) over existing walls, as seen in Plate 12.
Nowadays, 3DP in Construction strongly relies on cementitious mixtures, despite the attempts that have been made in using earth-based or supplementary cementitious materials (SCM). For instance, in the study conducted by Xu et al. (2017), the choice of a cementitious mortar was motivated by its physical properties (similar to stone), texture and colour, very close to the original, making this selection quite convenient.
However, for many cases, cementitious mortars will not be compatible with most of the ancient materials used, arising the need to develop new mixtures for 3DP with compatible materials. This places certain demands on currently used printers, which were mainly designed to extrude cementitious-based mortars and, therefore, require substantial adjustments (in terms of printhead, extrusion nozzle and pumping and control system), a time and labour-consuming exercise.
The design of new mixtures opens new doors in the field of 3DP by allowing the use of lighter, more resistant and sustainable materials. In the case of cultural heritage rehabilitation, the use of lime mortars should be tested and emphasised, as they are suitable for filling deteriorated areas of this type of buildings. At the same time, the addition of residues that are no longer useful promotes the circular economy and reduces excessive waste consumption.
Few efforts have been conducted regarding lime/gypsum or ceramic mortars in 3DP. Huang et al. (2021) introduced a heat-induced accelerator (HIA) in extrusion-based 3DP of gypsum. Due to the fast setting of the material, Powder Bed Binder Jetting is the preferable 3DP technique to use for gypsum. One of the best compositions contained gypsum, water, protein retarder and HIA and the other contained gypsum, water and protein retarder. When printed above high temperature (above 40°C), cylinder samples meet 3DP requirements, with acceptable collapse ratio and shape retention index, providing that with HIA, temperature could be used as a stimulus to control the setting on-demand for extrusion-based 3DP gypsum.
Palacios et al. (2020) developed 3DP mortars containing river sand, Portland cement, hydraulic lime, water and a plasticiser. Several doses were prepared with 7%, 10% and 12% of lime (concerning the % of cement). It was found that the inclusion of lime up to 10% did not affect the compressive strength of the mixtures; thus, only two dosages with 7% of lime and two more dosages without lime were selected (only differing in the amount of water). After conducting mechanical tests, specimens with lime had, on average, a 10% less compression strength at similar ages than non-lime mixtures.
Additionally, adobe, a very typical material in vernacular architecture, could not only be the great precursor of new 3D printed sustainable constructive solutions, but also an excellent material for the reinforcement of old structures.
The examples collected in chapters 4.2 and 4.3 were grouped in Table 5, in order to facilitate their understanding and comparison.
4.4 Future perspectives
Regardless of the key advances registered in the implementation of this technology in the construction sector, several difficulties associated, for example, to the structural reinforcement (Tay et al., 2017) of the developed structures or to their thermal performance (Pessoa et al., 2021) are already foreseen, with more to come. The potential integration of 3DP in the field of cultural heritage rehabilitation, addressed throughout this paper, is one of them. Table 6 summarises some of its benefits and major challenges.
Although clear, the advantages of the application of this technique will only be possible due to a strong collective effort between R&D (Research & Development) units, 3DP companies, heritage rehabilitation offices and groups responsible for establishing practices, methodologies and useful documentation for the correct preservation of this type of structures (as CEN/TC 346).
In parallel, given the pluridisciplinarity of this new area of knowledge, it will be of utmost importance to meticulously follow the various stages intrinsic to the restoration process, ensuring a correct compatibility between the different software used, an optimisation of the mixture to be extruded and the printing equipment (as seen in Figure 4), whilst respecting ethical issues associated with the preservation of the identity of the building under analysis.
It should be noted that if there is an insufficient level of rigour/effort in any of the stages associated with the process of reconstitution/reconstruction of heritage building elements, cases such as the recreation of Palmyra's Arch of Triumph, may occur, discrediting and denying the potential of this technology in this important sector.
The “printing process” phase is suitable for the repair of multiple size damages in several building elements, as seen in Plate 9 (right) and Figure 5. The 3D printable mixture developed (lime mortar, for example, as stated above) will have the ability to work as the filler material on the damaged cavities, as long as it its compatible with the existing support material.
While there is already some interest and primary research in linking these two thematic lines, further questions arise.
What changes are necessary in the composition of traditionally used lime-based mortars to make them extrudable?
Will these 3D printed mortars be compatible with the substrate to be restored?
Will these 3D printed mortars offer the same attributes as those traditionally conceived?
What adaptations will 3D printers undergo to be capable of printing lime-based mortars?
5. Conclusions
This article discusses the benefits and challenges of applying 3DP technology in the construction industry, particularly in the field of cultural heritage rehabilitation. The reflection carried out allowed to understand that its introduction in this sector leads to a great technological breakthrough, since it promotes the long-awaited automation of construction processes.
In spite of being studied for many years, some of the conservation and restoration techniques used in historical buildings are outdated and imprecise, which can lead to the reoccurrence of the same or other anomalies. Additionally, the current difficulty in finding materials that are the same or similar (in aesthetic and performance terms) to the former ones limits the effectiveness of the rehabilitation solutions employed.
3DP facilitates the creation of complex geometric shapes at a reduced cost, offering architects freedom in their projects and enabling the fabrication of unique elements. In contrast to traditional construction methods, the extrusion of material only where it is needed allows savings in its consumption, which provides an optimisation of construction components and reduces the industry's environmental impact. Its great potential does not only apply to the construction of new buildings or structures; it should be also capable of covering the restoration of ornamental elements in patrimonial buildings or the reconstruction of deteriorated parts of monuments, whether due to natural or human causes. With the use of mapping and 3DP technologies, the restoration or reconstruction work of these elements will become much more accessible, both laboriously and economically, as presented in many examples of Table 5. Also, the similarity between some of the materials used in historical buildings and 3DP may also serve as a kick-start for further investment and development in this area (natural stone and cementitious mortars; adobe and earth-based materials).
As shown in Table 1, anomalies like degradation of building elements or loss of mortar, both for coating and joints, can be rectified with 3DP, as seen in the examples presented in section 4.2. For this purpose, there will be two options: using well-developed robotic systems (Plate 2), since they provide a greater formal freedom and, in the future, will be able to print on any kind of non-horizontal surface or printing off-site and then assemble the printed piece in situ (such as the examples of Xu et al. (2017) or even Yeon et al. (2018)). Also, the use of mapping tools is crucial to reproduce reliable 3D digital models, an essential part in the creation of tailor-made ornaments via 3DP, while avoiding any kind of ethical implications.
In short, as far as the rehabilitation of cultural heritage is concerned, there is still a long trail to cover, not only related to the development of new mixtures with more interesting properties but also to the development of more specialised printers and their interoperability with the mapping tools previously presented.
Figures
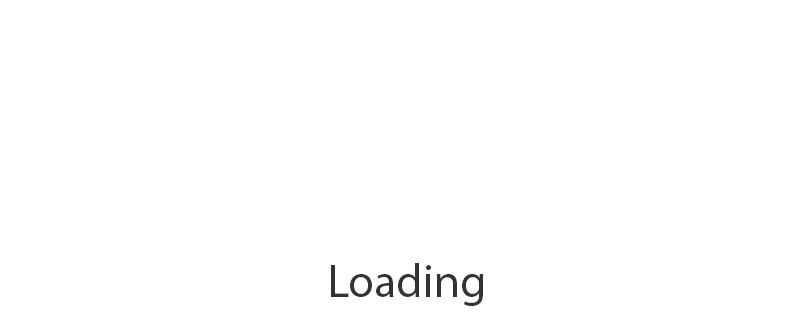
Figure 2
Contour Crafting technique (Zhang and Khoshnevis, 2013)
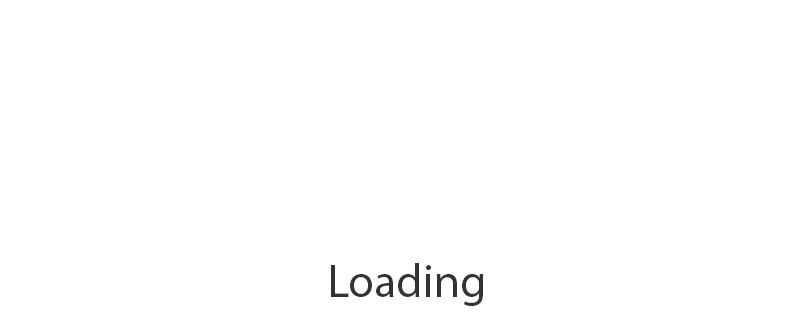
Plate 1
3D Concrete Printing facility at Loughborough University (left) (Lim et al., 2016)
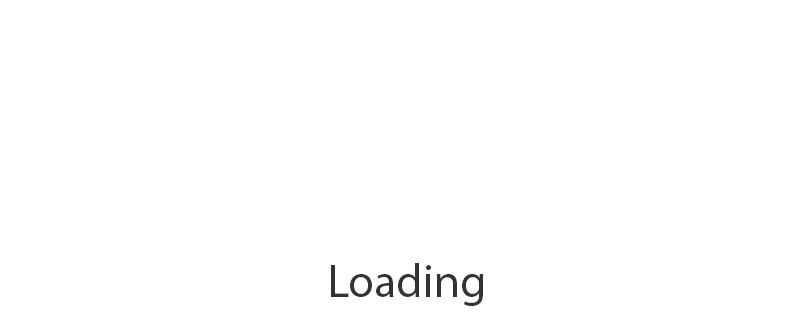
Plate 2
Robotic arm-based 3D printer (Rollakanti and Prasad, 2022)
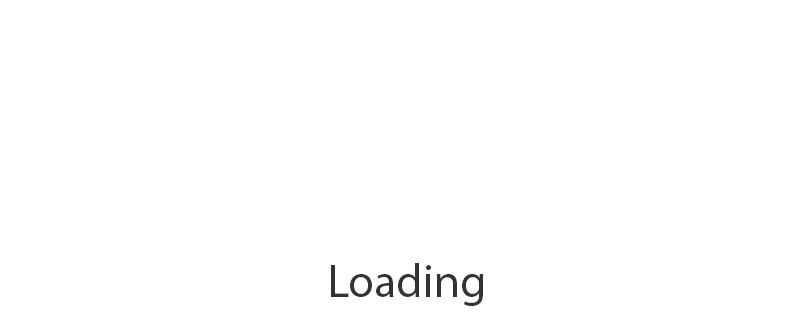
Plate 3
D-Shape 3D printer (left) and print head (right) (Lowke et al., 2018)
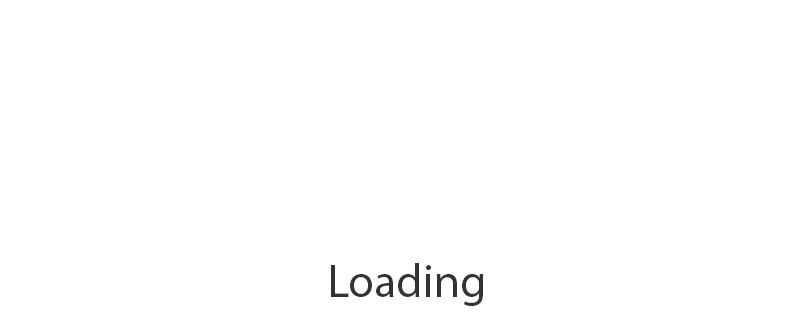
Plate 4
3D model (a), cross section with its containing shell (b) and printing process of “Radiolaria” (c) (Lowke et al., 2018)
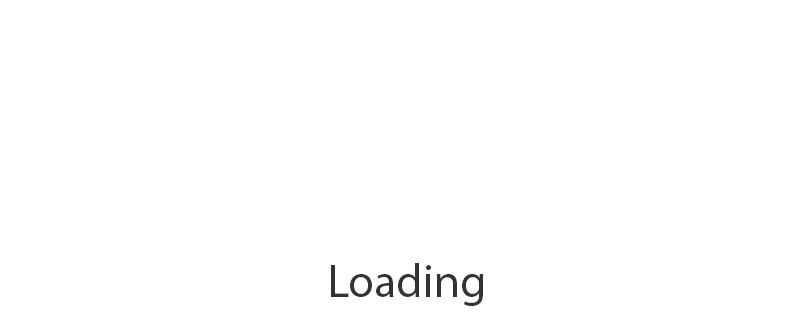
Plate 9
Phases of spall damage repair (Yeon et al., 2018): 3D printed plastic formwork (left), moulded concrete patch (centre) and final aspect of concrete patch attached on damaged surface (right)
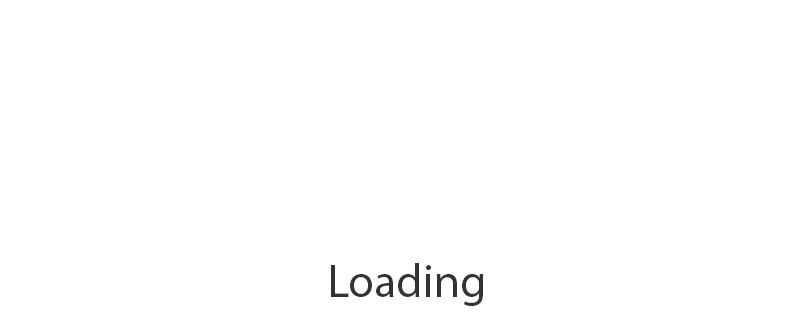
Figure 5
Different types of damaged cavities in heritage structures (Singh and Kumar, 2020)
Common anomalies and respective causes in heritage structures
![]() ![]() |
Fresh and hardened state requirements for 3D printable materials
Property | Definition | |
---|---|---|
Fresh State | Flowability/Pumpability | Capacity of the material to be smoothly transported from the mixer to the printing nozzle; it is affected by temperature and relative humidity |
Extrudability | Capacity of the material to continuously pass through the small pipes and nozzles at the printing nozzle without clogging up the system or interrupting the filament | |
Buildability/Shape Retention | Capacity of the material to retain its extruded shape and resistance throughout early ages, under self-weight and under the pressure from upper layers, without excessive deformation; it is possible by using high content of fine aggregates and sand | |
Open Time | Time interval in which the fresh material maintains a consistent flow rate for good extrudability (related to the change of flowability with time); the use of appropriate retarders can help controlling the open time | |
Hardened State | Interlayer Adhesion | The bond between two adjacent layers must be high to improve the structural integrity of the piece |
Drying Shrinkage | Due to the inexistence of formwork, the surface area that contacts with the air is considerable, allowing a high rate of water evaporation and accelerating drying shrinkage; this phenomenon highly increases the risk of cracking | |
Mechanical Strength | Compressive strength (usually tested at the ages of 3, 14 and 28 days), flexural strength (usually tested at the age of 7 days) and tensile bond strength between layers | |
Printability | When the material reaches all the desirable fresh and hardened properties, a suitable 3D printable material is achieved |
Source(s): Adapted from Lim et al. (2012), Ma et al. (2017), Ma et al. (2018), Le et al. (2012a), Buswell et al. (2018), Li et al. (2020)
Different types of 3D printable cementitious mortars
Developer(s) | Composition | Reference |
---|---|---|
TU/e | Cement, siliceous aggregate, limestone filler, rheology modifiers, polypropylene fibres, water | Bos et al. (2016) |
Guowei Ma, Zhijian Li, Li Wang | Cement, fly ash, silica fume, copper tailings, superplasticizer, water, polypropylene fibres | Ma et al. (2018) |
University of Nantes | Cement, limestone filler, sand, gravel, water, set accelerator | Furet et al. (2019) |
University of Southern California | Cement, sand, plasticiser, water | Hwang and Khoshnevis (2004) |
Loughborough University | Cement, silica fume, fly ash, superplasticizer, water, polypropylene fibres, retarder, sand | Le et al. (2012b) |
XtreeE SAS | Cement, crystalline silica, silica fume, limestone filler, sand, water | Gosselin et al. (2016) |
Most used components in 3D printable cementitious mortars
Paste | Additives | Fibres | Raw materials | |
---|---|---|---|---|
Water | Superplasticizer | Flax | PP* | Recycled glass |
Cement | Steel | Glass | ||
Accelerator | Sand | |||
Fly-ash | Carbon | Basalt | ||
Retarder | Cork | |||
Silica fume | PVA* | Copper |
Note(s): *PVA – Polyvinyl Acetate; PP – Polypropylene
Source(s): Craveiro et al. (2019)
Applications of 3DP for cultural heritage rehabilitation
Institution/Company | Project | Findings/Innovation | Material(s) | Manufacturing place | Source |
---|---|---|---|---|---|
Huazhong University of Science and Technology (China) | Reproduction of a damaged cup-shaped plinth | Work comprised 3D scanning, modelling and 3DP of a new plinth | Cement based-mortar for 3DP | Off-site; piece later assembled in situ | Xu et al. (2017) |
IDA and UNESCO | Recreation of Palmyra's Arch of Triumph | Work was based on 2D pictures of the original arch; two RobotMills robots printed several blocks; final structure has 6 m tall and 11 tonnes | Egyptian marble | Off-site; piece later assembled in situ | Jalabi (2016), Alexandrea (2017) |
3DION 3D network and voxeljet | Replication of Michael portal's patrons from Cologne Cathedral | 3D scanning of patrons and 3D model production; binder jetting technique to produce the replicas; post-processing (cleaning and application of epoxy resin) | PMMA powder | Off-site; piece later assembled in situ | Voxeljet (2020) |
Sismaitalia | Temporary replacement of grand Spada Palace capitals | Double printing of capitals (due to the dual printhead); after printed, a plaster finish was applied, followed by painting in the same colour | Polystyrene | Off-site; piece later assembled in situ | MASSIVit 3D (2018) |
Concr3de | Replication of Notre-Dame Cathedral's gargoyle | Available scans of the gargoyle were found on the Internet; a 3D printer with a precision of 0.1 mm was used to print the replicas | Mixture of limestone and ash | Off-site; piece later assembled in situ | deezen (2019) |
edg | Restoration of building façade elements | Creation of a 3D printer “formwork”, laser cut wire mesh and 3D printed stirrups (for reinforcement); the “formwork” is later filled with concrete | Cast concrete | Off-site; piece later assembled in situ | The Architect's Newspaper (2018) |
IAAC | Mataerial | 3DP of 3D curves, in any surface and direction | – | In situ | IAAC (2018) |
HOLCIM | 3D vertical printing | 3DP of specific mortars vertically and directly onto walls, for ornamental purposes | 3DP dry mortar (cementitious materials or natural hydraulic lime) | In situ | HOLCIM (2020) |
RWTH Aachen University (Germany) | Vertical extrusion of foam concrete | Vertical extrusion of foam concrete onto walls | Foam concrete | In situ | Lublasser et al. (2018) |
Nanyang Technological University (Singapore) | Vertical extrusion of cementitious materials with fly ash cenosphere | Vertical extrusion of cementitious materials with fly ash cenosphere onto walls | Cementitious materials with fly ash cenosphere | In situ | Bing et al. (2018) |
Analysis of the potential of 3DP in cultural heritage rehabilitation
Strengths |
| Challenges |
|
Opportunities |
|
References
3DPrint.com (2015a), “Compact Apis cor 3D printer constructs buildings in one day”, available at: https://3dprint.com/100229/apis-cor-3d-printer-buildings/ (accessed 14 March 2021).
3DPrint.com (2015b), “Concrete plans: CyBe's berry Hendriks describes Plans to 3D Print with mortar”, available at: https://3dprint.com/35727/cybe-berry-hendriks-concrete/ (accessed 22 March 2022).
Alexandrea, P. (2017), 3D technology in the restoration of history.
Alhumayani, H., Gomaa, M., Soebarto, V. and Jabi, W. (2020), “Environmental assessment of large-scale 3D printing in construction: a comparative study between cob and concrete”, Journal of Cleaner Production, Vol. 270, 122463.
Appleton, J. (2003), Rehabilitation of Old Buildings: Pathologies and Intervention Technologies (In Portuguese), Edições ORION, Amadora.
Armesto-González, J., Riveiro-Rodríguez, B., González-Aguilera, D. and Rivas-Brea, M.T. (2010), “Terrestrial laser scanning intensity data applied to damage detection for historical buildings”, Journal of Archaeological Science, Vol. 37, pp. 3037-3047.
ASTM International (2013), Standard Terminology for Additive Manufacturing Technologies, ASTM International, West Conshohocken, PA.
Bhatla, A., Choe, S.Y., Fierro, O. and Leite, F. (2012), “Evaluation of accuracy of as-built 3D modeling from photos taken by handheld digital cameras”, Automation in Construction, Vol. 28, pp. 116-127.
Bing, L., Mingyang, L., Kah Fai, L., Shunzhi, Q. and Ming Jen, T. (2018), Develop cementitious materials incorporating fly ash cenophere for spray-based 3D printing.
Bos, F., Wolfs, R., Ahmed, Z. and Salet, T. (2016), “Additive manufacturing of concrete in construction: potentials and challenges of 3D concrete printing”, Virtual and Physical Prototyping, Vol. 11, pp. 209-225.
Buswell, R.A., Leal de Silva, W.R., Jones, S.Z. and Dirrenberger, J. (2018), “3D printing using concrete extrusion: a roadmap for research”, Cement and Concrete Research, Vol. 112, pp. 37-49.
CEN (2011), Conservation of Cultural Property – Main General Terms and Definitions, CEN, Brussels.
CIB (2010), Guide for the Structural Rehabilitation of Heritage Buildings, CIB Commission W023, Ottawa, ON.
Craveiro, F., Duarte, J.P., Bartolo, H. and Bartolo, P.J. (2019), “Additive manufacturing as an enabling technology for digital construction: a perspective on construction 4.0”, Automation in Construction, Vol. 103, pp. 251-267.
Dalenogare, L.S., Benitez, G.B., Ayala, N.F. and Frank, A.G. (2018), “The expected contribution of Industry 4.0 technologies for industrial performance”, International Journal of Production Economics, Vol. 204, pp. 383-394.
deezen (2019), “Concr3de proposes using 3D printing to rebuild Notre-Dame”, available at: https://www.dezeen.com/2019/04/24/3d-printing-notre-dame-cathedral-concr3de/ (accessed).
Dini, E. (2020), “D-Shape”, available at: https://d-shape.com/ (accessed 22 2020).
Döndüren, M.S. and Sişik, O. (2017), “Materials, used in historical buildings, analysis methods and solutions puroposals”, E3S Web of Conferences, Vol. 19, 03019.
El-Hakim, S., Gonzo, L., Voltolini, F., Girardi, S., Rizzi, A., Remondino, F. and Whiting, E. (2007), “Detailed 3D modelling of castles”, International Journal of Architectural Computing, Vol. 5, pp. 200-220.
Factum Foundation (2016), “IDA Palmyra arch copy”, available at: https://www.factumfoundation.org/pag/236/ (accessed 30 May 2022).
FIEC (2017), “Digitalisation, construction 4.0 and BIM”, available at: https://www.fiec.eu/priorities/digitalisation-construction-40-and-bim (accessed).
France 24 (2019), “Can Notre-Dame rise from the ashes with 3D printing?”, available at: https://www.france24.com/en/20190427-paris-france-notre-dame-rebuild-reconstruction-concr3de-3d-printing-macron (accessed).
Fröhlich, C. and Mettenleiter, M. (2004), “Terrestrial laser scanning-new perspectives in 3D surveying”, International Archives of Photogrammetry, Remote Sensing and Spatial Information Sciences, Vol. XXXVI-8/W2, pp. 7-13.
Furet, B., Poullain, P. and Garnier, S. (2019), “3D printing for construction based on a complex wall of polymer-foam and concrete”, Additive Manufacturing, Vol. 28, pp. 58-64.
García, D., Plazaola, X., Vegas, I. and Areizaga, P. (2017), “BIM for pre-demolition and refurbishment inventories and waste information management”, HISER International Conference 2017: Advances in Recycling and Management of Construction and Demolition Waste, Delft.
Gosselin, C., Duballet, R., Roux, P., Gaudillière, N., Dirrenberger, J. and Morel, P. (2016), “Large-scale 3D printing of ultra-high performance concrete – a new processing route for architects and builders”, Materials and Design, Vol. 100, pp. 102-109.
HOLCIM (2020), “3D printing dry mortar range - A first for HOLCIM”, available at: https://www.holcim.com/lafargeholcim-spain-introduces-our-first-dry-mortar-range-3d-printing (accessed 20 February 2022).
Huang, J., Duan, B., Cai, P., Manuka, M., Hu, H., Hong, Z., Cao, R., Jian, S. and Ma, B. (2021), “On-demand setting of extrusion-based 3D printing gypsum using a heat-induced accelerator”, Construction and Building Materials, Vol. 304, 124624.
Hwang, D. and Khoshnevis, B. (2004), “Concrete wall fabrication by Contour crafting”, 21st International Symposium Automation and Robotics in Construction, Jeju Island.
IAAC (2016), “TerraPerforma - 3D printed performative wall”, available at: https://iaac.net/project/terraperforma/ (accessed 16 November 2020).
IAAC (2018), “Mataerial”, available at: https://iaac.net/project/mataerial/ (accessed 21 February 2022).
ISO/ASTM (2015), Additive Manufacturing - General Principles - Terminology, ISO/ASTM, Geneva.
Jalabi, R. (2016), “Replica of Syrian arch destroyed by Isis unveiled in New York City”, available at: https://www.theguardian.com/us-news/2016/sep/20/palmyra-arch-syria-new-york?CMP=fb_gu&utm_medium=website&utm_source=archdaily.com (accessed).
Khoshnevis, B. (2004), “Automated construction by contour crafting - related robotics and information technologies”, Automation in Construction, Vol. 13 No. 1, pp. 5-19, doi: 10.1016/j.autcon.2003.08.012.
Khunti, R. (2018), “The problem with printing Palmyra: exploring the ethics of using 3D printing technology to reconstruct heritage”, Studies in Digital Heritage, Vol. 2, pp. 1-12.
Kumar, V., Singh, R., Ahuja, I.P.S. and Hashmi, M.S.J. (2020), “On technological solutions for repair and rehabilitation of heritage sites: a review”, Advances in Materials and Processing Technologies, Vol. 6, pp. 146-166.
Lagüela, S., Díaz-Vilariño, L., Armesto, J. and Arias, P. (2014), “Non-destructive approach for the generation and thermal characterization of an as-built BIM”, Construction and Building Materials, Vol. 51, pp. 55-61.
Le, T., Austin, S., Lim, S., Buswell, R.A., Gibb, A. and Thorpe, A. (2012a), “Mix design and fresh properties for high-performance printing concrete”, Materials and Structures, Vol. 45, pp. 1-12.
Le, T.T., Austin, S.A., Lim, S., Buswell, R.A., Law, R., Gibb, A.G.F. and Thorpe, T. (2012b), “Hardened properties of high-performance printing concrete”, Cement and Concrete Research, Vol. 42, pp. 558-566.
Leica Geosystems (2016), Leica ScanStation P30/P40 Product Specifications, Leica Geosystems, Heerbrugg.
Li, Z., Hojati, M., Wu, Z., Piasente, J., Ashrafi, N., Duarte, J.P., Nazarian, S., Bilén, S.G., Memari, A.M. and Radlińska, A. (2020), “Fresh and hardened properties of extrusion-based 3D-printed cementitious materials: a review”, Sustainability, Vol. 12, p. 5628.
Lim, S., Buswell, R.A., Le, T., Wackrow, R., Austin, S., Gibb, A. and Thorpe, A. (2011), “Development of a viable concrete printing process”, Proceedings 28th International Symposium Automation and Robotics in Construction, Seoul, South Korea.
Lim, S., Buswell, R.A., Le, T.T., Austin, S.A., Gibb, A.G.F. and Thorpe, T. (2012), “Developments in construction-scale additive manufacturing processes”, Automation in Construction, Vol. 21, pp. 262-268.
Lim, S., Buswell, R.A., Valentine, P.J., Piker, D., Austin, S.A. and De Kestelier, X. (2016), “Modelling curved-layered printing paths for fabricating large-scale construction components”, Additive Manufacturing, Vol. 12, pp. 216-230.
Liudmila, C. and Balkiz, Y. (2019), “Chapter 14 - bridging sustainable construction technologies and heritage: novel approach to the conservation of the built environment”, in TAM, V.W.Y. and LE, K.N. (Eds), Sustainable Construction Technologies, Butterworth-Heinemann, Oxford.
Lourenço, P.B. (2002), “Computations on historic masonry structures”, Progress in Structural Engineering and Materials, Vol. 4, pp. 301-319.
Lowke, D., Dini, E., Perrot, A., Weger, D., Gehlen, C. and Dillenburger, B. (2018), “Particle-bed 3D printing in concrete construction – possibilities and challenges”, Cement and Concrete Research, Vol. 112, pp. 50-65.
Lublasser, E., Adams, T., Vollpracht, A. and Brell-Cokcan, S. (2018), “Robotic application of foam concrete onto bare wall elements - analysis, concept and robotic experiments”, Automation in Construction, Vol. 89, pp. 299-306.
Ma, G., Wang, L. and Ju, Y. (2017), “State-of-the-Art of 3D printing technology of cementitious material - an emerging technique for construction”, Science China Technological Sciences, Vol. 61, pp. 475-495.
Ma, G., Li, Z. and Wang, L. (2018), “Printable properties of cementitious material containing copper tailings for extrusion based 3D printing”, Construction and Building Materials, Vol. 162, pp. 613-627.
MASSIVit 3D (2018), “Nineteenth century palace restored using MASSIVIT 3D printing”, available at: https://massivit3d.com/nineteenth-century-palace-restored-using-massivit-3d-printing/ (accessed).
Moropoulou, A., Labropoulos, K.C., Delegou, E.T., Karoglou, M. and Bakolas, A. (2013), “Non-destructive techniques as a tool for the protection of built cultural heritage”, Construction and Building Materials, Vol. 48, pp. 1222-1239.
Palacios, J., Paredes, M., Castillo, T. and Paredes, O. (2020), “Mortar for 3D printers using river sand, Portland cement and hydraulic lime”, Scientific Review Engineering and Environmental Sciences, Vol. 29, p. 399.
Perrot, A., Rangeard, D. and Courteille, E. (2018), “3D printing of earth-based materials: processing aspects”, Construction and Building Materials, Vol. 172, pp. 670-676.
Pessoa, S., Guimarães, A.S., Lucas, S.S. and Simões, N. (2021), “3D printing in the construction industry - a systematic review of the thermal performance in buildings”, Renewable and Sustainable Energy Reviews, Vol. 141, 110794.
Prieto, A.J., Silva, A., de Brito, J., Macías-Bernal, J.M. and Alejandre, F.J. (2017), “The influence of pathological situations on churches' functionality: an approach based on historical records”, International Journal of Architectural Heritage, Vol. 11, pp. 566-587.
Rollakanti, C.R. and Prasad, C.V.S.R. (2022), “Applications, performance, challenges and current progress of 3D concrete printing technologies as the future of sustainable construction – a state of the art review”, Materials Today: Proceedings.
Rushing, T.S., Stynoski, P.B., Barna, L.A., Al-Chaar, G.K., Burroughs, J.F., Shannon, J.D., Kreiger, M.A. and Case, M.P. (2019), “Chapter 7 - investigation of concrete mixtures for additive construction”, in Sanjayan, J.G., Nazari, A. and Nematollahi, B. (Eds), 3D Concrete Printing Technology, Butterworth-Heinemann.
SAHC (2019), “Secondary materials in mortars for use in historical buildings (based on the thesis of Menard Kilumile, Beijing University)”, available at: https://blog.msc-sahc.org/secondary-materials-in-mortars-for-use-in-historical-buildings/ (accessed 22 February 2022).
Singh, R. and Kumar, R. (2020), “Solid polymer waste materials for repairing of heritage composite structure: an additive manufacturing approach”, in Hashmi, S. and Choudhury, I.A. (Eds), Encyclopedia of Renewable and Sustainable Materials, Elsevier, Oxford.
Tavares, A., Costa, A. and Varum, H. (2011), Building Rehabilitation and Maintenance Manual: Intervention Guide (In Portuguese), INOVADOMUS, Portugal, Aveiro.
Tay, Y.W.D., Panda, B., Paul, S., Noor Mohamed, N.A., Tan, M.J. and Leong, K.F. (2017), “3D printing trends in building and construction industry: a review”, Virtual and Physical Prototyping, Vol. 12, pp. 261-276.
The Architect's Newspaper (2018), “edg creates customizable 3D-printed concrete molds”, available at: https://www.archpaper.com/2018/05/edg-creates-customizable-3d-printed-concrete-molds/#gallery-0-slide-0 (accessed).
UNESCO (2009), “Cultural heritage”, available at: http://uis.unesco.org/en/glossary-term/cultural-heritage (accessed 22 February 2022).
voxeljet (2020), “3DION and voxeljet completed a replica of the Cologne cathedral”, available at: https://www.voxeljet.com/case-studies/art-and-design/replicas-for-the-cologne-cathedral/ (accessed).
WASP (2017), “3D printed houses for a renewed balance between environment and technology”, available at: https://www.3dwasp.com/en/3d-printed-houses-for-a-renewed-balance-between-environment-and-technology/ (accessed 18 February 2022).
Wines, J. and Jodidio, P. (2000), Green Architecture, Taschen, Koln and New York.
Wingtra (2021), “Drone photogrammetry vs. LIDAR: what sensor to choose for a given application”, available at: https://wingtra.com/drone-photogrammetry-vs-lidar/ (accessed 29 November 2021).
Wolfs, R.J.M. and Salet, T.A.M. (2016), “Potentials and challenges in 3D concrete printing”, 2nd International Conference on Progress in Additive Manufacturing, Singapore, 16-19 May 2016, pp. 8-13.
Xu, J., Ding, L. and Love, P.E.D. (2017), “Digital reproduction of historical building ornamental components: from 3D scanning to 3D printing”, Automation in Construction, Vol. 76, pp. 85-96.
Yeon, J., Kang, J. and Yan, W. (2018), “Spall damage repair using 3D printing technology”, Automation in Construction, Vol. 89, pp. 266-274.
Yossef, M. and Chen, A. (2015), “Applicability and limitations of 3D printing for Civil structures”, Conference Autonomous and Robotic Construction of Infrastructure, 2-3 June 2015, Ames, Iowa, pp. 237-246.
Zhang, J. and Khoshnevis, B. (2013), “Optimal machine operation planning for construction by Contour Crafting”, Automation in Construction, Vol. 29, pp. 50-67.
Acknowledgements
The authors would like to thank all the owners of external figures who have kindly authorised their republication in this document. Manuel Jesus would like to thank FCT for financial support through the doctoral grant UI/BD/151533/2021. Finally, this work was financially supported by Base Funding - UIDB/04708/2020 of the CONSTRUCT Instituto de I&D em Estruturas e Construções - funded by national funds through the FCT/MCTES (PIDDAC).