Abstract
Purpose
This study aims to determine the influence of bruise damage generated from the impact test on the physical, chemical and nutritional responses of tomato fruit.
Design/methodology/approach
The impact loading was applied from different heights. The impact energies for 20, 40 and 60 cm drop heights were 129.59, 259.18 and 388.77 mJ, respectively. The injured samples were kept for 48 hours at low (10 °C) and ambient (22 °C) storage temperatures. Weight loss, firmness, color, total soluble solids (TSS), lycopene and carotenoids were measured before the impact test (day 0) and after 48 hours of the impact and storage.
Findings
The drop height of 60 cm and storage at 22 °C showed the highest values in the bruised area. The impact from the 60 cm drop height significantly reduced weight, lightness, yellowness, hue, firmness, lycopene and carotenoids, particularly at 22 °C storage condition. Redness (a*) and color index (CI) showed a remarkable increase (p < 0.05) at 22 °C on tomatoes affected from the highest impact level (388.77 mJ) after 48 hours of storage. No pronounced significance was seen between TSS and drop heights. This study has confirmed that tomato bruising for a short-term storage period induces physiological changes at different storage temperature conditions.
Originality/value
The study can confirm the crucial role of inappropriate handling in increasing fresh produce loss within short-term storage. Also, this research can be considered as a guideline for transporters, handlers, processors, distributors and horticulture researchers in the fresh produce supply chain during postharvest operations.
Keywords
Citation
Pathare, P.B., AL-Dairi, M. and Al Mahdouri, A. (2023), "Influence of drop test impact on the physiochemical attributes of tomatoes", Arab Gulf Journal of Scientific Research, Vol. ahead-of-print No. ahead-of-print. https://doi.org/10.1108/AGJSR-10-2022-0213
Publisher
:Emerald Publishing Limited
Copyright © 2023, Pankaj B. Pathare, Mai AL-Dairi and Adil Al Mahdouri
License
Published in Arab Gulf Journal of Scientific Research. Published by Emerald Publishing Limited. This article is published under the Creative Commons Attribution (CC BY 4.0) licence. Anyone may reproduce, distribute, translate and create derivative works of this article (for both commercial and non-commercial purposes), subject to full attribution to the original publication and authors. The full terms of this licence may be seen at http://creativecommons.org/licences/by/4.0/legalcode
1. Introduction
Tomato is very popular due to its important nutritional values and is considered to be healthy since it contains β-carotene and lycopene, which reduce the incidence of cardiovascular diseases and cancer (Pathare & Al-Dairi, 2021). Consumers prefer high-quality fresh produce, which are assessed on their appearance, taste, firmness and freshness (Al-Dairi, Pathare, & Al-Yahyai, 2021). With a long marketing chain, and postharvest operations like transportation, picking and handling, fresh produce are subjected to different external forces that cause bruising (Sun, Pessane, Pan, & Wang, 2021). Bruising is the most common form of mechanical damage, which can cause different physical and chemical quality alterations in tomatoes (Buccheri & Cantwell, 2014). Also, Xia et al. (2020) reported that the most crucial reason for bruising and mechanical damages during the postharvest supply chain is extreme impact forces generated from dropping against the surface of the package. As reviewed by Al-Dairi, Pathare, Al-Yahyai, and Opara (2022), bruising is the most known type of mechanical damage, which is resulted from dynamic loading because of excessive vibration and impact. Besides, bruise damage is a failure of subcutaneous tissue, which does not include the rapture of the fresh produce skin. Improper or rough handling, improper packaging and unsuitable supervision during handling can increase the occurrence of bruise damage.
Opara and Pathare (2014) documented the influence of bruising on quality attributes in the horticulture industry. Bruising can decrease the quality of fresh produce, causing losses to the growers and producers due to low sale prices (Kim, Lim, Kim, & Choi, 2020). Bruise damage in fruits and vegetables increases the rate of metabolism and produces a higher loss of moisture content, which therefore increases weight loss (Hussein, Fawole, & Opara, 2019). Also, bruising can influence the interior quality, change of physiological processes and increase postharvest decay of fresh horticultural produce (Pathare, Al-Dairi, & Al-Mahdouri, 2021; Scherrer-Montero, Dos Santos, Andreazza, Getz, & Bender, 2011). Bruise damage accelerates physiological damage leading to spoilage (Sun et al., 2021), internal browning, faster ripening and quality losses (Al-Dairi, Pathare, & Al-Mahdouri, 2022; Opara & Pathare, 2014). Damage due to bruising accelerated the firmness reduction of tomatoes (Buccheri & Cantwell, 2014), D’Anjou pears (Pathare & Al-Dairi, 2021) and kiwifruit (Xia et al., 2020). Also, it caused fruit browning on apples (Ergun, 2017), lycopene content reduction in tomatoes (Buccheri & Cantwell, 2014) and internal changes like total soluble solids (TSS) losses in pomegranate (Hussein, Fawole, & Opara, 2020; Pathare, Al-Dairi, Al-Yahyai, & Al-Mahdouri, 2022).
Bruise damage is a measure of exterior loading response and mostly depends on different elements like temperature, maturity, variety, size, shape, etc. (Buccheri & Cantwell, 2014). Pathare and Al-Dairi (2021) stated that temperature is a post-climacteric factor that impacts the bruising of fresh produce. Temperature increases the bruising damage and accelerates the tissue flexibility of fresh fruits and vegetables. Hence, this study aimed to recognize the effect of bruising by simulating the method of drop impact test on tomato physical, chemical and nutritional attributes, and investigate its effect on two storage temperature conditions for 48 hours of storage.
2. Materials and methods
2.1 Tomato sample acquisition, drop test, storage and bruise area susceptibility measurements
Tomatoes “Miral” variety at light red maturity stage packed in wooden containers were obtained from the market and directly delivered to Postharvest Technology Laboratory, Sultan Qaboos University, Oman. A total of 21 fruits were selected to provide a fairly uniform color, firmness and weight (65.45 ± 8.12), and to ensure that the samples were free from cracks and other defects. Bruising damage to each tomato fruit was made by the drop impact test method as described by Pathare and Al-Dairi (2021) (Figure 1A). The setup consisted of a 66.05 g stainless steel ball, which was dropped once from three different height levels of 20, 40 and 60 cm (representing the low, medium and high drop levels, respectively) through the hollow Polyvinyl chloride (PVC) guiding pipe. After the first rebound, the steel ball was caught by hand to avoid multiple impacts on to a tomato sample. A total of six fruit were impacted by each drop height (n = 6 per drop height). The bruise of each tomato sample was marked to enable bruise measurements and recognition.
After the impact test, tomato fruit of different drop heights were divided equally and stored at 10 and 22 °C. A total of three replications were included per drop height per storage temperature. The fruit were stored for 2 days (48 hours) to allow bruise appearance on the damaged surface of the tomato fruit. The impact energy (Ei, mJ) per drop height was determined following Equation (1) (Pathare & Al-Dairi, 2021).
Besides, the equivalent of tomato fruit drop height (Heq, cm) to each Ei was measured using Equation (2) (Hussein et al., 2020).
After 48 hours of storage, the bruise diameter including major and minor widths (w1 and w2) were determined by utilizing a digital caliper (Model: Mitutoyo, Mitutoyo Corp., Japan) (Figure 1A). The bruise area (BA, mm2) (semi-oblate) was calculated based on Equation (3) (Opara & Pathare, 2014). Also, bruise area susceptibility (BAS, mm2/J) was calculated by using Equation (4) below:
During the experiment, the temperature was checked by using a temperature meter (Model: TES 13604, TES Electrical Corp., Taiwan). Different physical, chemical and nutritional analyses were conducted for tomatoes before and after the impact. A total of three tomato fruit were evaluated before the impact test for day-0 analysis. The layout of the experiment has been shown in Figure 1B.
2.2 Determination of physical quality analysis
2.2.1 Weight and firmness loss (%)
The tomato fruit was weighed before impact and after 48 hours of storage by applying an electric weight balance (Model: GX-4000, Japan) with an accuracy of ±0.01 g. A total of three tomatoes for each treatment were weighed to identify the weight loss %. The % of weight loss was determined as the change of initial weight Wi of tomato fruit and weight Wf after 48 hours of storage divided by the initial weight as shown in Equation (5).
The firmness of three tomato fruit from each treatment was determined by penetrating two opposite sides per tomato surface using a digital fruit firmness tester (Model: FHP-803, L.L.C., USA). Firmness reduction was presented in percentage (Equation 6).
2.2.2 Color
A total of 15 external color readings were recorded from three tomato fruit per group before and after the impact (90 readings per day) using a computer vision system (RGB image acquisition system) as explained by Al-Dairi, Pathare, and Al-Mahdouri (2021). The red, green and blue (RGB) values obtained from the system were analyzed using ImageJ software (v. 1.53, National Institute of Health, MD, USA). Later, the original RGB values were converted to CIEL*a*b* color space, which is the highly applied color space in most fresh fruits and vegetable studies. Chroma* that indicates color intensity (Equation 7), a hue* that refers to color purity (Equation 8), and color index (CI) that identifies red color development in tomato (Equation 9) were also calculated (Pathare, Opara, & Al-Said, 2013).
2.3 Determination of chemical and nutritional quality analysis
2.3.1 Total soluble solids (TSS)
Tomato juice was extracted by homogenizing tomatoes (n = 3) per group for one minute using a food mixture (Model: LM2201, Moulinex, China). A muslin cloth was used to filter the extracted juice. Drops of the filtered juice were added directly to the prism of the digital refractometer (Model: PR-32 α, ATAGO Co., Ltd, Japan). TSS were expressed as Brix (Al-Dairi, Pathare, & Al-Yahyai, 2021).
2.3.2 Total lycopene and carotenoids
Lycopene and carotenoid contents were obtained by utilizing a spectrophotometer method as defined by Al-Dairi, Pathare and Al-Yahyai (2021). One gram of the tomato juice was extracted using 14 ml n-hexane: acetone (3:2 v/v) by utilizing an Eppendorf centrifuge (Model: Sanyo MSE Harrier 18/80, Sanyo, Tokyo, Japan) at a relative centrifugal speed of 10,000 for 10 minutes at 4 °C. The obtained supernatant was topped up to 25 ml with extraction solution. Later, Ultraviolet/Visible/Near Infrared (UV/VI/NIR) spectrophotometer (Model: Lambd900, PERKIN ELMER, USA) was used to identify the absorbance at (502 nm). Total lycopene and carotenoid pigments of tomatoes per treatment were determined prior to the impact drop test and after the storage period. The results were presented in %.
2.4 Statistical analysis
A two-factorial analysis of variance was performed to identify the effect of the independent variables including drop height (20, 40 and 60 cm) and storage temperature (10 and 22 °C) on the dependent variables, namely, weight loss, firmness, color, TSS, total lycopene and carotenoids at 5% significance level (p < 0.05) by applying SPSS 20.0 (International Business Machine Crop., USA). Tukey’s range Honestly Significant Difference (HSD) test was performed to compare the mean of the main treatments. The resulting data were presented in mean ± standard deviation (S.D). In the current study, five linear regression models were developed to examine the influence of the independent variable on some dependent variables like weight loss, BAS, redness, firmness, TSS and lycopene. Also, the determination coefficient (R2) was reported to verify the accuracy of the models.
3. Results and discussions
3.1 Bruise impact, equivalent drop height of the fruit and bruise damage area susceptibility
The impact energies generated from low, medium and high-impact levels were 129.59, 259.18 and 388.77 mJ, respectively. Generally, the sample of fruit impacted from 60 cm drop height absorbed the greatest energy. The impact energies obtained from the current study are in the range of threshold energies that can generate damage to the tomato fruit as revealed by Ghaffari, RezaGhassemzadeh, Sadeghi, and Alijani (2015). It was possible to determine the specific drop height of tomato fruit that is equivalent to the recorded impact energy (Ei) by using the average mass of fruit bruised from different heights.
The Heq of tomatoes could be an important parameter in practical applications since fruit fall can be considered as a typical impact that occurs during postharvest harvesting, handling and transport operations. Therefore, increasing farmers’ and handlers’ knowledge to understand the impact levels has the potential to reduce damages to fresh produce (Hussein, Fawole, & Opara, 2017). Results in Table 1 showed that tomato fruit with the lowest impact level from the low (20 cm) drop height had the lowest equivalent drop height (Heq = 18.97 cm), whereas tomato fruit with the highest impact level from the high (60 cm) drop height had the highest equivalent drop height (Heq = 41.99 cm).
The existence of a bruise can take more than 12 hours or even longer to appear, which is based on the severity of the damage (Xia et al., 2020). A significant effect was observed between the BA of tomatoes, and both drop height level (p = 0.02159) and storage temperature conditions (p < 0.00001) after 48 hours of storage (Figure 2A). The BA increased from the lower impact level (20 cm drop height) to the higher one (60 m drop height) across all storage conditions. The drop height of the impactor from 60 cm (388.77 mJ) and storage at 22 °C showed a high rise in the BA (419.76 mm2) of tomatoes after 48 hours of storage. At the end of storage, the minimum value of the BA was found for the drop height of 20 cm (129.59 mJ) and storage at 10 °C. This indicates that storage at cold temperature conditions could be an effective way to reduce the incidence of bruise damage. The BAS was also affected by drop height (p < 0.0468) and storage temperature (p < 0.0494) (Table 1). Tomatoes bruised from the highest level and stored at 22 and 10 °C recorded the highest BAS with 1.46 and 1.13 mm2 mJ−1, respectively. The least value was observed on those bruised from the lowest impact level and stored at 10 °C (0.83 mm2 mJ−1) (Figure 2B).
Generally, the study recorded that increasing both drop height and storage temperature increased the BA and BAS of tomatoes after 48 hours of storage. Pathare and Al-Dairi (2021) and Hussein et al. (2019) recorded the same findings on tomato and pomegranate, respectively, where increasing the drop height significantly increased the bruise measurements of the fruit. Besides, Tabatabaekoloor (2013) reported that the more drop height, the more potential energy can occur which accelerates contact intensity, consequently, increasing the BA of fruit. Similarly, Hussein et al. (2019) found a significant increase in BA from 20 to 60 cm drop in height in pomegranate. Storage at both conditions had a positive effect on the BA. However, storage at 22 °C exhibited a higher increment in BA. According to Ahmadi (2012), high storage temperature can increase the incidence of bruising in fruit where enzymes are still active leading to cell wall degradation and stiffness as well. Also, the results support the findings of Pathare and Al-Dairi (2021) who stated that decreasing storage temperature declined bruise damage in tomatoes. Bugaud, Ocrisse, Salmon and Rinaldo (2014) examined the influence of storage conditions on bananas and revealed that decreasing the storage temperature from 18 to 13 °C decreased bruise damage. Also, Pathare and Al-Dairi (2022) recorded a 163.45 mm2 BA in high-impact bruised banana fruit stored at ambient temperature. Table 3 shows the final BA (model 1), which contains all the independent variables (drop height and storage temperature). For model 1, the plot of predicted and measured BA is shown in Figure 3. A strong fit with R2 = 0.920 was recorded between the predicted BA and the measured BA values.
3.2 Effect on weight and firmness loss
Weight loss (%) of tomatoes after 48 hours of storage is shown in Figure 4A. This study has successfully revealed that weight loss increased significantly with drop height (bruising) (p = 0.00549) and storage temperature condition (p = 0.00001) after storage (Table 2). Weight loss was highly pronounced in tomatoes (1.99%) subjected to an impact from the highest impact level (60 cm drop height and 388.77 mJ) stored at 22 °C. Tomatoes stored at room temperature and impacted by the medium (40 cm drop height and 259.18 mJ) and low (20 cm drop height and 129.59 mJ) impact levels lost their weight by 1.26 and 1.24%, respectively. The weight loss was lower in tomatoes subjected to an impact from low (0.44 %), medium (0.60 %) and high (0.66 %) drop heights after 48 hours of storage at 10 °C. The current study showed that weight loss increased more rapidly in tomatoes stored at 22 °C.
The greatest loss % in tomatoes weight observed in highly bruised could be attributed to possible permeability and damage to the tissue cell wall, which consequently leads to a higher transpiration rate during storage (Hussein et al., 2020). Al-Dairi and Pathare (2021) found that storage at 22 °C can accelerate the percentile of weight loss in tomatoes due to the increase in water dehydration, respiration and transpiration processes. Hussein et al. (2020) revealed a reduction in weight loss at low-temperature storage of more than 8-fold lower than ambient temperature storage in bruised fruit due to the low rate of metabolic processes that occur at cold temperatures. Figure 3B illustrates the predicted weight loss% plotted versus the measured weight loss% in relation to impact level and storage temperature (model 2). A good fit (R2 = 0.847) was found between the predicted and measured weight loss% (Table 3).
The current study found that the firmness of tomato fruit significantly varied between drop height level (p = 0.01845) and storage temperature condition (p = 0.00224) after storage as presented in Table 2. After 48 hours at both storage temperatures, the firmness subjected to an impact from a height of low (20 cm), medium (40 cm) and high (60 cm) levels dramatically decreased. On the last day of storage at 22 °C, the highest % of reduction in firmness was observed in tomatoes impacted by 60 cm drop height (388.77 mJ) with 3.67% loss followed by those damaged from drop heights of 40 and 20 cm with 2.74 and 2.11% loss, respectively (Figure 4B). Storage at 10 °C showed 2.06, 1.83 and 0.84% firmness reduction in tomato fruit impacted by an impact from 60, 40 and 20 cm drop heights, respectively (Figure 4B).
The most critical outcome of this test is that as the BA increases, the firmness reduces after 48 hours, particularly at room temperature. Also, Al-Dairi, Pathare, and Al-Mahdouri (2021) confirmed that high-temperature storage conditions affect the firmness of fresh produce because of the enzyme activity, resulting in the cell wall and polysaccharide degradation of fresh produce. Different studies revealed that elevating the drop height level reduced the firmness of pears (Pathare & Al-Dairi, 2021) and apples (Azadbakht, Mahmoodi, & Vahedi Torshizi, 2019). For the firmness value prediction model (3), the plot of measured and predicted firmness is shown in Figure 3C. A moderate fit was found between the predicted and the measured firmness (R2 = 0.640).
3.3 Effect on the color attributes
In this part of this study, a significant (p < 0.05) effect of drop height and storage temperature was observed in all color parameters for 48 hours of storage, except for chroma*, where drop height did not show a significant variation (p > 0.05) on bruised tomato (Table 2) particularly at a lower temperature. With increasing drop height and storage temperature, the values of tomato lightness (L*) reduced and darkness increased (Figure 5A). Lightness decreased, respectively, by 0.10, 0.09 and 0.07% on tomatoes impacted from a drop height of 60 cm (high level), 40 cm (medium level) and 20 cm (low level) at room temperature after 48 hours of storage period. At the end of storage, the impacted fruits at the lowest drop height level and storage at 10 °C showed the lowest reduction in tomato lightness with 0.05%. In contrast, the redness (a*) value increased for 48 hours of storage at both storage conditions in all tomatoes subjected to an impact of different heights (Figure 5B). The increase in redness (a*) was more obvious in tomatoes (0.30%) stored at 22 °C and bruised from the highest impact level (60 cm drop height ∼388.77 mJ) and lowest (0.03%) in tomatoes impacted by the impactor from the lowest level (40 cm drop height ∼25921 mJ). Yellowness (b*) decreased after 48 hours duration under all tested treatments (Figure 5C). The percentage of the reduction was significantly higher (0.21%) in tomatoes bruised from the highest impact (60 cm) and stored at 22 °C and was lower (0.06%) in tomatoes stored at 10 °C and subjected to an impact from low impact level (20 cm).
Tomatoes stored at 22 °C increased chroma* in all bruised tomatoes after 48 hours of storage compared to those stored at 10 °C, which showed fluctuated changes in chroma* between treated tomatoes (Figure 5D). The results indicated a delayed reduction in hue° of tomato impacted by the lowest impact level (20 cm) stored at 10 °C (39.01–36.31 °C). Storage at 22 °C showed a loss in hue° from 39.01° on day 0 to 25.95° after 48 hours of storage (Figure 5E). Besides, a high increment in CI was observed on tomatoes impacted by the highest impact level and stored at 22 °C (Figure 5F).
A similar trend of decrease in L* was detected on bruised tomatoes at 20 °C (Lee, Kim, Kim, & Park, 2005). Pathare and Al-Dairi (2021) found that the color attributes of bruised pears were highly influenced by bruise damage. In this study, storage at 22 °C accelerated the changes of all color parameters, which were initially started due to bruising. For storage temperature, room temperature can reduce the L* value due to tomato darkening resulting from carotenoids synthesis (Kim et al., 2020) and increases the a* value because of chlorophyll degradation and lycopene synthesis (Al-Dairi et al., 2021). The hue° reduction in tomatoes is associated with the rate of a biochemical reaction that highly occurs at room temperature compared to cold storage temperature (Cherono, Sibomana, & Workneh, 2018). Kabir et al. (2020) recorded that the storage of tomatoes at 10 °C is the most recommended storage temperature used to preserve color attributes like redness. The plot of predicted a* values versus measured a* values is shown in Figure 6 (model 4). A strong fit was observed between the measured and predicted a* values with the determination coefficient (R2) of 0.872 (Table 3).
3.4 Effect on total soluble solids lycopene and carotenoids
The TSS values presented in Figure 7A indicated no pronounced (p = 0.37867) effect of bruising resulting from different drop heights on the TSS of tomatoes. However, TSS contents were significantly (p = 0.04460) affected by storage temperature after storage (Table 2). The initial value of TSS was 4.40. The highest total soluble (TSS) content was observed in tomatoes after storage at 22 °C and impacted by low, medium and high-impact levels with values of 4.53, 4.50 and 4.56 °Brix, respectively. Storage at 10 °C delayed the increment of TSS contents. The results of the study support the findings of Hussein et al. (2020), where no significant effect was found between TSS content and impact level in pomegranate fruit. The increase observed in TSS contents at 22 °C was attributed due to the transformation of starch to simpler sugar resulting from the active enzymes at this temperature condition (Al-Dairi et al., 2021).
The total lycopene content was affected by both drop height (p = 0.02764) and storage temperature (p = 0.01788) (Table 2). When comparing the total lycopene for tomatoes impacted by an impact from 20, 40 and 60 cm drop heights, the highest % of reduction in tomato lycopene content was 1.25% and observed on tomatoes impacted by the highest impact level (60 cm drop height ∼388.77 mJ) after 48 hours of storage at room temperature (Figure 7B). At the end of storage at 10 °C, the impact from low (20 cm), medium (40 cm) and high (60 cm) drop heights showed 0.33, 0.50 and 0.96% reduction in lycopene. Overall, the impact from the lowest level and storage at cold temperature slowed the % of lycopene content reduction compared to stored tomato at room temperature and impacted from the highest impact level after short-term storage (48 hours). Buccheri and Cantwell (2014) observed a higher reduction in the lycopene content of bruised tomatoes compared to non-bruised tomatoes. A similar trend of reduction was also observed in the carotenoid content of tomatoes at both storage temperature conditions impacted by the low, medium and high drop heights (Figure 7C). Storage temperature and drop height statistically affected (p < 0.05) the carotenoid content after 48 hours of storage (Table 2). Tomatoes bruised from 20 cm drop height (low impact) and stored at 10 °C showed less than 0.2% reduction in carotenoids compared to those bruised from 60 cm drop height (high impact) and stored at 22 °C with more than 0.4% reduction % after 48 hours of storage. Table 3 presents the results of the lycopene model (5) and all independent variables. The coefficient of determination (R2) was 0.760 between the predicted and measured values of lycopene. Figure 6B demonstrates the relationships between the predicted and measured values gained for lycopene value from the linear regression model.
4. Conclusions
The present study showed that the BA and BAS of tomatoes increased as drop height (impact level) increased after 48 hours of storage. The increment was highly observed in tomatoes stored at 22 °C compared to those stored at 10 °C. Among the three common drop heights, the impact from the 60 cm drop height (388.77 mJ) greatly increased the percentage of loss in weight, firmness, lightness, color purity, yellowness, lycopene and carotenoids contents and increased the percentage of redness and CI at room temperature after 48 hours of storage. However, the lowest impact level and storage at 10 °C delayed the increase of BA and BAS in tomatoes and all assessed physical and nutritional quality attributes. The TSS of tomatoes were significantly affected by storage temperature conditions. However, no pronounced effect was observed between TSS and the drop height. In general, this can help to improve the knowledge on dealing with tomatoes during postharvest handling, transportation and other postharvest operations to reduce all possible damages due to bruising. Further studies are required to be highly focused on the effect of multiple drop heights that are facing tomato fruit during real postharvest handling to study their subsequent effect on the fruit and figure out more solutions to prevent losses.
Figures
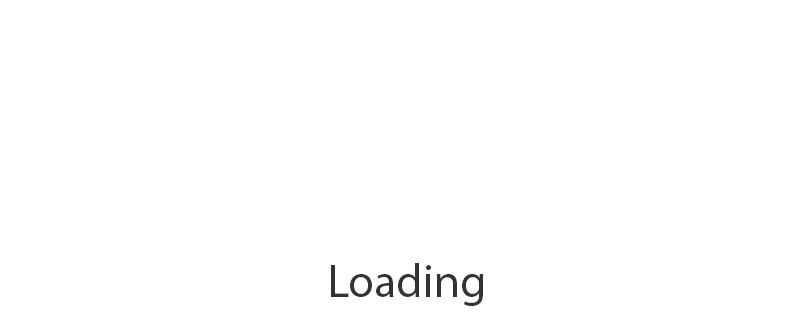
Figure 2.
(A) Bruise area (BA) and (B) bruise area susceptibility (BAS) values of tomato subjected to impact from three drop heights; 20, 40, 60 cm after 48 h of storage at 10 and 22 °C. Error bars express the standard deviation of the mean values. Bars with different letters are significantly different
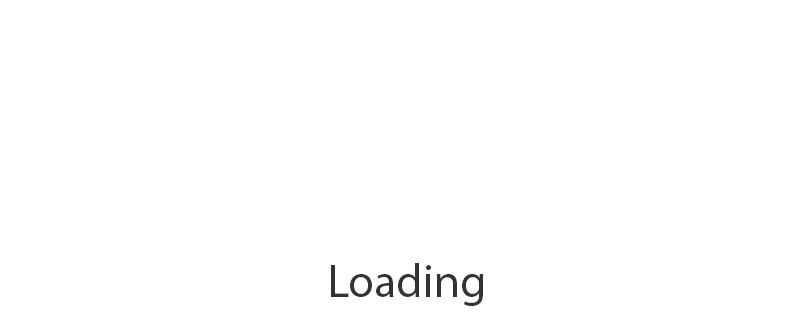
Figure 5.
Color measurements, (A) L* reduction %, (B) a* increment %, (C) b* reduction %, (D) chroma*, (E) hue*, and (F) color index values of tomato subjected to impact from three drop heights; 20, 40, 60 cm after 48 h of storage at 10 and 22 °C. Error bars express the standard deviation of the mean values. Bars with different letters are significantly different
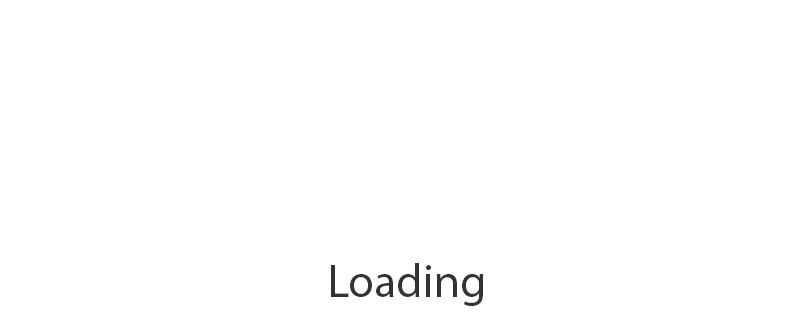
Figure 7.
(A) Total soluble solids, (B) lycopene loss %, and (C) carotenoids loss % values of tomato subjected to impact from three drop heights; 20, 40, 60 cm after 48 h of storage at 10 and 22 °C. Error bars express the standard deviation of the mean values. Bars with different letters are significantly different
Estimated tomato fruit drop height that is required to produce bruise damage during the impact of three energies
Drop height (cm) | Tomato fruit mass (g) | Impact threshold (mJ) for bruise damage | Equivalent drop height (cm) |
---|---|---|---|
20 | 69.61 ± 6.86 | 129.59 | 18.97 |
40 | 74.96 ± 10.43 | 259.18 | 35.24 |
60 | 94.36 ± 9.19 | 388.77 | 41.99 |
Source(s): Table by the authors
The statistical analysis of BA, BAS, L*, a*, b*, chroma*, hue*, CI, total soluble solids, lycopene and carotenoids of tomato subjected to impact from three drop heights, 20, 40 and 60 cm, after 48 hours of storage at 10 and 22 °C
Bruise measurement | Statistical test | A | B | A |
---|---|---|---|---|
BA | p value | 0.0001 | 0.0215 | 0.2200 |
df | 2 | 1 | 2 | |
F-value | 40.3383 | 6.9670 | 1.7220 | |
BAS | p value | 0.0468 | 0.0494 | 0.5042 |
df | 2 | 1 | 2 | |
F-value | 3.9931 | 3.9021 | 0.7252 | |
Weight loss% | p value | 0.0054 | 0.0001 | 0.8164 |
df | 2 | 1 | 2 | |
F-value | 8.2847 | 52.853 | 0.2062 | |
Lightness (L*) | p value | 0.0022 | 0.0184 | 0.2930 |
df | 2 | 1 | 2 | |
F-value | 10.5801 | 7.4227 | 1.3619 | |
Redness (a*) | p value | 0.0221 | 0.0070 | 0.6948 |
df | 2 | 1 | 2 | |
F-value | 9.7451 | 10.5285 | 0.3753 | |
b* | p value | 0.0039 | <0.0001 | 0.0511 |
df | 2 | 1 | 2 | |
F-value | 9.0623 | 117.7903 | 3.8470 | |
Hue* | p value | 0.0008 | 0.0013 | 0.9917 |
df | 2 | 1 | 2 | |
F-value | 13.5460 | 17.3290 | 0.0083 | |
Chroma* | p value | 0.0319 | 0.0001 | 0.3081 |
df | 2 | 1 | 2 | |
F-value | 4.6540 | 32.2990 | 1.3000 | |
CI | p value | 0.3400 | 0.0013 | 0.392 |
df | 2 | 1 | 2 | |
F-value | 1.1820 | 17.3000 | 1.0140 | |
p value | 0.0280 | 0.0001 | 0.7793 | |
df | 2 | 1 | 2 | |
F-value | 4.8857 | 32.4225 | 0.2545 | |
TSS | p value | 0.3788 | 0.4460 | 0.3483 |
df | 2 | 1 | 2 | |
F-value | 1.0540 | 5.0284 | 1.1529 | |
Lycopene | p value | 0.0276 | 0.0178 | 0.49006 |
df | 2 | 1 | 2 | |
F-value | 6.9212 | 10.4406 | 0.8051 | |
Carotenoids | p value | 0.0286 | 0.0173 | 0.76605 |
df | 2 | 1 | 2 | |
F-value | 6.8007 | 10.5884 | 0.2780 |
Note(s): A; drop height, factor B; Storage temperature
Source(s): Table by the authors
Linear regression equations of dependent variables in relation to the independent variables
Model | Equation | R2 |
---|---|---|
1 | BA = −2.900 + 102.008DH + 49.073ST | 0.846 |
2 | WL% = −0.862 + 0.315DH + 0.923ST | 0.847 |
3 | FL% = 3.518 | 0.640 |
4 | a* = 19.184 + 1.123DH + 4.704ST | 0.872 |
5 | Lycopene = −0.442 + 0.303DH + 0.431ST | 0.760 |
Note(s): BA; bruise area, WL%; weight loss, FL%; firmness, DH; drop height, ST; storage temperature
Source(s): Table by the authors
References
Ahmadi, E. (2012). Bruise susceptibilities of kiwifruit as affected by impact and fruit properties. Research in Agricultural Engineering, 58(3), 107–113.
Al-Dairi, M., & Pathare, P. B. (2021). Kinetic modeling of quality changes of tomato during storage. Agricultural Engineering International: CIGR Journal, 23(1), 183–193.
Al-Dairi, M., Pathare, P. B., & Al-Mahdouri, A. (2021). Effect of storage conditions on postharvest quality of tomatoes: A case study at market-level. Journal of Agricultural and Marine Sciences, 26(1), 13–20.
Al-Dairi, M., Pathare, P. B., & Al-Mahdouri, A. (2021). Impact of vibration on the quality of tomato produced by stimulated transport. Paper presented at the IOP Conference Series: Earth and Environmental Science.
Al-Dairi, M., Pathare, P. B., & Al-Mahdouri, A. (2022). The contribution of impact damage to the quality changes of stored banana fruits. Biology and Life Sciences Forum, 16(1), 1–8.
Al-Dairi, M., Pathare, P. B., & Al-Yahyai, R. (2021). Effect of postharvest transport and storage on color and firmness quality of tomato. Horticulturae, 7(7), 163.
Al-Dairi, M., Pathare, P. B., & Al-Yahyai, R. (2021). Quality changes kinetic of tomato during postharvest transportation and storage. Journal of Food Process Engineering, 44(10), e13808. doi:10.1111/jfpe.13808.
Al-Dairi, M., Pathare, P. B., Al-Yahyai, R., & Opara, U.L. (2022). Mechanical damage of fresh produce in postharvest transportation: Current status and future prospects. Trends in Food Science & Technology, 124(1), 195–207.
Azadbakht, M., Mahmoodi, M. J., & Vahedi Torshizi, M. (2019). Effects of different loading forces and storage periods on the percentage of bruising and its relation with the qualitative properties of pear fruit. International Journal of Horticultural Science and Technology, 6(2), 177–188.
Buccheri, M., & Cantwell, M. (2014). Damage to intact fruit affects quality of slices from ripened tomatoes. LWT, 59(1), 327–334.
Bugaud, C., Ocrisse, G., Salmon, F., & Rinaldo, D. (2014). Bruise susceptibility of banana peel in relation to genotype and post-climacteric storage conditions. Postharvest Biology and Technology, 87, 113–119.
Cherono, K., Sibomana, M., & Workneh, T. S. (2018). Effect of infield handling conditions and time to pre-cooling on the shelf-life and quality of tomatoes. Brazilian Journal of Food Technology, 21, e2017016.
Ergun, M. (2017). Physical, physiochemical and electrochemical responses of ‘Galaxy’ Apples to mild bruising. European Journal of Horticultural Science, 82(5), 244–250.
Ghaffari, H., RezaGhassemzadeh, H., Sadeghi, M., & Alijani, S. (2015). Some physical, mechanical and chemical properties of tomato fruit related to mechanical damage and bruising models. Paper presented at the Biological Forum.
Hussein, Z., Fawole, O. A., & Opara, U. L. (2017). Investigating bruise susceptibility of pomegranate cultivars during postharvest handling. African Journal of Rural Development, 2(1), 33–39.
Hussein, Z., Fawole, O. A., & Opara, U. L. (2019). Bruise damage susceptibility of pomegranates (Punica granatum, l.) and impact on fruit physiological response during short term storage. Scientia Horticulturae, 246, 664–674.
Hussein, Z., Fawole, O. A., & Opara, U. O. (2020). Effects of bruising and storage duration on physiological response and quality attributes of pomegranate fruit. Scientia Horticulturae, 267, 109306.
Kabir, S. N., Rasool, K., Lee, W. -H., Cho, S. -I., & Chung, S. -O. (2020). Influence of delayed cooling on the quality of tomatoes (Solanum lycopersicum L.) stored in a controlled chamber. AIMS Agriculture and Food, 5(2), 272.
Kim, D. S., Lim, J. H., Kim, S., & Choi, J. H. (2020). Agreement between visual and model-based classification of tomato fruit ripening. Transactions of the ASABE, 63(3), 667–674.
Lee, H. J., Kim, T. -C., Kim, S. J., & Park, S. J. (2005). Bruising injury of persimmon (Diospyros kaki cv. Fuyu) fruits. Scientia Horticulturae, 103(2), 179–185.
Opara, U. L., & Pathare, P. B. (2014). Bruise damage measurement and analysis of fresh horticultural produce—a review. Postharvest Biology and Technology, 91, 9–24.
Pathare, P. B., & Al-Dairi, M. (2021). Bruise damage and quality changes in impact-bruised, stored tomatoes. Horticulturae, 7(5), 113.
Pathare, P. B., & Al-Dairi, M. (2021). Bruise susceptibility and impact on quality parameters of pears during storage. Frontiers in Sustainable Food Systems, 5, 658132.
Pathare, P. B., & Al-Dairi, M. (2022). Effect of mechanical damage on the quality characteristics of banana fruits during short-term storage. Discover Food, 2(1), 4.
Pathare, P. B., Opara, U. L., & Al-Said, F. A. (2013). Colour measurement and analysis in fresh and processed foods: A review. Food and Bioprocess Technology, 6(1), 36–60.
Pathare, P. B., Al-Dairi, M., & Al-Mahdouri, A. (2021). Bruise damage susceptibility and fruit quality assessment of pear. The Open Agriculture Journal, 15(1), 82–90.
Pathare, P. B., Al-Dairi, M., Al-Yahyai, R., & Al-Mahdouri, A. (2022). Effect of impact-bruising and storage on quality physiological changes of Omani pomegranate. Paper presented at the V International Symposium on Pomegranate and Minor Mediterranean Fruits 1349.
Scherrer-Montero, C., Dos Santos, L., Andreazza, C., Getz, B., & Bender, R. (2011). Mechanical damages increase respiratory rates of citrus fruit. International Journal of Fruit Science, 11(3), 256–263.
Sun, Y., Pessane, I., Pan, L., & Wang, X. (2021). Hyperspectral characteristics of bruised tomatoes as affected by drop height and fruit size. LWT, 141, 110863.
Tabatabaekoloor, R. (2013). Engineering properties and bruise susceptibility of peach fruits (Prunus persica). Agricultural Engineering International: CIGR Journal., 15(4), 244–252.
Xia, M., Zhao, X., Wei, X., Guan, W., Wei, X., Xu, C., & Mao, L. (2020). Impact of packaging materials on bruise damage in kiwifruit during free drop test. Acta Physiologiae Plantarum, 42(7), 1–11.
Acknowledgements
This study was financially supported by Sultan Qaboos University (Grant No. IG/AGR/SWAE/19/03) and (Grant No. IG/AGR/Dean/18/01).